
고체산화물 연료전지용 삼중 전도성 산화물 환원극: 리뷰


Copyright © The Korean Society for Precision Engineering
This is an Open-Access article distributed under the terms of the Creative Commons Attribution Non-Commercial License (http://creativecommons.org/licenses/by-nc/3.0) which permits unrestricted non-commercial use, distribution, and reproduction in any medium, provided the original work is properly cited.
Abstract
Solid oxide fuel cells (SOFCs) are at a technological level close to commercialization, which could be enabled by new material research. Especially, not only an electrolyte, but also a cathode material becomes very important to further increase electrochemical performance, due to the effort to lower operating temperature of SOFCs to intermediate range (400-600oC) to take advantage of high and low temperature operation. Unfortunately, this trend inevitably results in demand for new cathode materials with high oxygen reduction reaction activity, as well as high mechanical durability. Recently, ceramic materials which conduct oxygen ion, proton, and electron, thereby called ‘triple conducting oxide’ are being highlighted, due to their excellent material properties, to be used for cathodes of SOFCs. This paper reviews the three representative triple-conducting oxides, which were already used and tested in SOFC operating conditions.
Keywords:
Triple-conducting oxide, Solid oxide fuel cell, Oxygen reduction reaction, Cathode, Perovskite키워드:
삼중 전도성 산화물, 고체 산화물 연료전지, 산소 환원 반응, 환원극, 페로브스카이트1. 서론
연료전지는 화학 에너지를 전기화학반응을 통해 전기에너지로 직접 변환시켜주는 장치로서 친환경성, 고 에너지변환 효율, 고에너지밀도, 크기별 확장 대응성 (Scalability), 다양한 종류의 연료 사용 가능성 등의 장점을 가지고 있어 미래 대체에너지로서 각광받고 있다.1-4 특히 고분자 전해질막 연료전지(Polymer Electrolyte Membrane Fuel Cell, PEMFC), 고체산화물 연료전지(Solid Oxide Fuel Cell, SOFC), 인산 연료전지(Phosphoric Acid Fuel Cell, PAFC), 용융 탄산염 연료전지(Molten Carbonate Fuel Cell, MCFC) 등은 이미 상용화되어 수송용, 발전용 등으로 사용되고 있으며, 해마다 계속하여 보급률이 증가하는 중에 있다.5
연료전지의 종류는 전해질을 기준으로 나뉘며, 여러 연료전지들 중 SOFC는 고체산화물(세라믹) 전해질이 높은 이온전도도를 띠게 해야 하는 특성상 PEMFC, PAFC, MCFC 등 대비 가장 높은 작동 온도 영역대(> 700oC)를 보인다. 이러한 특성으로 인해 전기화학 촉매로서 백금과 같은 고가의 귀금속을 사용하지 않아도 되는 점, 탄화수소계 연료를 개질 없이 직접 사용 가능한 등의 장점이 있을 수 있으나, SOFC 기술을 시스템화 및 패키징하는 과정에서 단열의 어려움, 밀봉재 등이 고온을 견뎌야 하는 데에서 발생하는 재료적 한계 등의 문제점을 야기한다.2,6,7
따라서 SOFC의 작동온도를 400 - 600oC으로 낮출 경우, 상술한 SOFC의 장점은 모두 취하면서 고온작동으로 인해 야기되는 문제를 해결할 수 있어, 이를 위한 많은 연구가 진행중이다.1,8,9 이러한 연구는 접근 방법에 따라 구조적 개선과 재료적 개선의 2가지로 나눌 수 있다.
구조적 개선은 고체산화물 연료전지의 구조적인 개선 및 변화를 통해 성능을 높이는 것을 뜻하며, 대표적으로 전해질을 박막화하여 전해질 내의 이온전도에 의해 발생되는 옴저항(Ohmic Resistance) 및 이에 따른 옴손실(Ohmic Loss)을 줄이는 방법이 있다. 이 접근법은 SOFC의 대부분의 성능 손실이 세라믹 전해질의 낮은 이온전도도에 기인하는 것에 대한 대응으로서, 다양한 물리적 및 화학적 박막 증착법을 통해 세라믹 전해질의 두께를 나노미터 수준으로 낮추는 방법이 있다. 그러나 이 방법은 세라믹 구조의 결정성 저하 및 이로 인한 반응성 및 이온전도도 저하, 기계적 강도 저하, 고가 공정으로 인한 연료전지 가격 상승 등의 문제점이 있다.1,9-38
재료적 개선은 기존에 알려지지 않은 새로운 물질을 개발하여 도입하는 것을 말하며, SOFC 전해질의 대표적인 예로 YSZ ((ZrO2)0.92(Y2O3)0.08) 전해질을 도핑된 산화 세륨계(Doped Ceria) 물질로 바꾸어 이온전도도를 높이려는 연구,39,40 LSC (La0.6Sr0.4CoO3-δ) 환원극을 LSCF (La0.6Sr0.4Co0.8Fe0.2O3-δ) 또는 BSCF (Ba0.5Sr0.5Co0.2Fe0.8O3-δ) 등의 공동 도핑(Co-Doping) 페로브스카이트(Perovskite) 물질로 바꾸는 것 등이 있다.41,42 이러한 시도들은 구조적 개선 접근 방법과 달리, 물질적 특성에서 야기되는 결점을 근본적으로 해결할 수 있어 구조적 개선 대비 근본적인 해결책일 수 있다. 상술한 페로브스카이트 물질들은 특히 SOFC 연구분야에서 최근 많은 연구가 중인데, 이는 페로브스카이트 구조를 통해 수많은 물질적 특성을 조절 및 구현할 수 있기 때문이다. 페로브스카이트 물질은 ABO3와 같은 원자 구조를 가지는 금속 산화물을 지칭하며, SOFC용 물질은 보통 BaZrO3 또는 BaCeO3계 페로브스카이트에 도핑 또는 공동 도핑을 하여 수소이온, 산소이온, 전자 전도도를 조절 또는 부여한다.41,43,44
특히 SOFC의 경우 구조적 개선 및 재료적 발전에 의해 작동 온도가 중저온영역으로 낮아지게 되면 PEMFC와 같은 저온 연료 전지에서 관찰되는 고 활성화손실(Activation Loss)을 동일하게 갖게 되는 문제가 있어, 이를 해결하기 위해 높은 산소 환원 반응성(Oxygen Reduction Reaction, ORR)을 보이는 물질이 활발히 연구중이다. 일반적으로 도핑된 BaCeO3계열은 높은 촉매활성도를 보이나 화학적 안정성이 낮아 장시간 구동에 취약하며, 도핑된 BaZrO3계 물질은 화학적 안정성은 놓으나 촉매활성도가 충분하지 않은 것으로 알려져있다.39,43,44
본 연구에서는 이러한 SOFC 전극용 물질 중 삼중 전도성(Triple-Conducting Oxide, TCO) (수소이온, 산소이온, 전자 전도성)물질에 대한 최근의 보고를 간단히 재조명한다. 연료전지에서 반응이 일어나는 삼상계면(Triple-Phase Boundary, TPB)은 반응물, 전극, 전해질이 만나는 곳으로서, 이곳을 늘리기 위해서 전극은 Fig. 1(a)와 같이 MIEC (Mixed Ion and Electron Conductor)의 형태로 만들어왔다. 그러나 최근 수소이온 전도 SOFC에 관한 연구가 활발해짐에 따라 이에 최적화된 환원극의 설계가 필요해졌고, 산소이온 전도형 MIEC의 경우 적합하지 않다는 주장이 여럿 보고된 바 있다.45,46 이 경우 Fig. 1(b)와 같이 산소이온, 전자와 함께 수소이온을 같이 전도시킬 경우 산소이온은 MIEC 표면에서 확산을 통해 전달되며, 동시에 전해질로부터 전도된 수소이온도 전극에서 전도 가능케 함으로써 TPB를 최대화하여 성능을 극대화할 수 있다. 또한 이는 단순히 수소이온 전도 SOFC 뿐만 아니라 산소이온 전도 SOFC에서도 성능 및 내구도 측면 모두에서 우수한 성능을 보인다.47–50 이러한 TCO 환원극을 일반 페로브스카이트 구조인 BCFZY (BaCo0.4Fe0.4Zr0.1Y0.1O3-δ)와 BSCF (Ba0.5Sr0.5Co0.8Fe0.2O3-δ), 계층화 페로브스카이트 구조인 NBSCF (NdBa0.5Sr0.5Co1.5Fe0.5O5+δ), 그 외 일반 계층 구조(Layered Structure)인 LNCO (LiNi0.8Co0.2O2) 세가지로 나눠보도록 한다.
2. 페로브스카이트 환원극
BaCo0.4Fe0.4Zr0.1Y0.1O3-δ는 수소이온 전도 SOFC의 환원극을 위해 설계됐으나,47 이후 일반 산소이온 전도 SOFC에서도 높은 성능과 안정성을 보이는 것으로 확인됐다.51 처음에는 BaCo0.4Fe0.4Zr0.2O3-δ의 형태로 수소이온 전도 SOFC의 환원극으로 실증됐으나 여기에 Y를 도핑함으로서 발견되게 됐다.52,53 수소이온 전도 산화물로 매우 잘 알려진 BZY (BaZrxY1-xO3-δ)을 전해질로 사용할 때 기존의 페로브스카이트 환원극에서 성능이 충분히 나오지 않아 BZY에 수소이온 전도성과 산소이온 전도성을 가지도록 하기 위해 전이금속류를 공동 도핑하여 합성된다. BZY 자체는 높은 수소이온 전도도를 보이나 전기전도도가 매우 낮아, 이를 보완하기 위해 ABO3의 B 위치에 Co와 Fe를 대량으로 공동 도핑하며, 이로 인해 산소이온과 전자 모두 전도 가능하게 된다. 특히 첫 BCFZY 보고에서는 BZY와 달리 졸-겔 공정으로 손쉽게 합성되는 것으로 보고하였다. NiO-BZY|BZY|BCFZY 구조로 500oC에서 수소/공기 공급시 455 mW/cm2, 메탄/공기 공급시 142 mW/cm2의 성능을 보였다.47 본 연구는 특히 메탄 연료를 직접 사용하여 1,400시간 작동을 하였을 때도 성능이 저하되지 않는 것을 보여 성능뿐만 아니라 장기 안정성도 매우 훌륭함을 보였으며, 수소이온 전도 SOFC에 산소 이온 전도 SOFC에서 주로 사용되는 환원극을 도입할 경우 호환이 되지 않아 성능이 저하되는 문제를 해결하였음을 알 수 있다. Fig. 2는 수소이온 전도 SOFC에 적용한 BCFZY의 1,400시간 작동 후의 전자현미경 사진과 장기간 성능 변화를 보여준다.
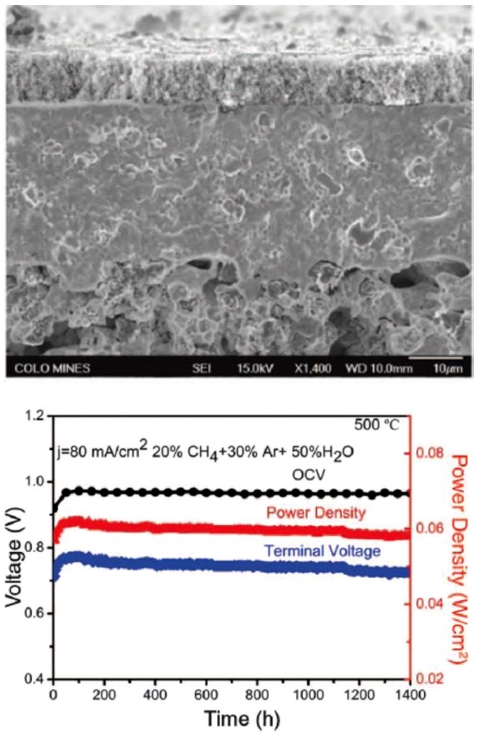
(Top) Cross-sectional image after 1400 h of operation and (Bottom) long-term durability test result of proton-conducting ceramic fuel cell with Ni-BZY|BZY|BCFZY. (Adapted with permission from Ref. 47)
본 BCFZY는 수소이온 전도 SOFC 뿐만 아니라 산소이온 전도 SOFC에 적용하여도 높은 전기화학적 성능과 안정성을 보였다.51 NiO-GDC (Ce1-xGdxO3-δ)|GDC|BCFZY의 구조로 수소/공기공급시 500, 450, 400, 350oC 작동시 각각 970, 640, 320, 130 mW/cm2의 최대전력밀도를 보였으며 2,500시간의 안정적 성능을 보였다. 또한 반복적 가열/냉각 테스트에서도 우수한 내구성을 보여 수소이온 전도 SOFC 뿐만 아니라 일반 산소이온 전도 SOFC에서도 적용 가능함을 보인 바 있다. BCFZY는 이 외에도 다양한 연료를 사용하는 연료전지에서도 조건에 상관 없이 매우 우수한 성능 및 장기 내구성을 보이는 것이 실험적으로 입증되었다.
BSCF 환원극도 삼중 전도성 물질로 알려져있으며, LSCF (La0.6Sr0.4Co0.8Fe0.2O3-δ) 물질과 함께 페로브스카이트 환원극으로 SOFC에 매우 많이 쓰인 바 있다.41,42,52 그러나 BSCF는 LSCF와 달리 삼전도 물질로 추정된 바 있으며,50 500oC와 600oC의 작동 온도 및 수소/공기 공급시 각각 0.4W/cm2와 1.1 W/cm2의 최대 전력밀도를 보여 타 SOFC용 환원극 개발에 있어 비교대상으로 계속 재조명되고 있다.41
3. 계층화된 페로브스카이트 환원극
연료전지 환원극에 사용된 계층화된 페로브스카이트 (Layered Perovskite) TCO 환원극으로서 NBSCF 물질이 있다.54 BCFZY와 같은 페로브스카이트 물질이나 이는 계층화된(Layered) 페로브스카이트로서 AA'B2O5+δ 구조를 가지며 여기서 A는 보통 Pr, Nd, Sm, Gd와 같은 3가 란타나이드 이온이 위치하게 된다. A' 자리는 Ba나 Sr, B는 전이금속이온이 위치하게 되며, AO|BO2와 A'Oδ|BO2 두 층이 교대로 쌓아올려져 계층화 페로브스카이트가 이뤄지게 된다. 본 구조를 통해 전기 전도도 완화(Electrical Conductivity Relaxation) 현상과 이온 교환 깊이 프로파일(Ion Exchange Depth Profile) 결과에 따라 저온에서도 매우 높은 전기전도도와 물질 표면에서의 산소 물질 전달, 그리고 매우 높은 산소이온 확산성을 보이게 된다.55–57 이러한 구조에 의해 고이온전도도를 야기하는 산소이온 전도 경로가 생기게 되는 것으로 알려져있다.54,58,59 뿐만 아니라 이러한 계층화된 페로브스카이트는 다른 페로브스카이트 물질 대비 고 수소이온 전도도를 가진 것으로 알려진 바 있어 수소이온 전도 SOFC용 환원극으로 높은 가능성이 제시되고 있다.50
본 NBSCF 환원극을 BCZYYb (BaCe0.7Zr0.1Y0.1Yb0.1O3-δ) 전해질 및 NiO-BCZYYb 도성 합금(Cermet)에 적용한 연료전지의 성능이 Fig. 3에 나와있다. 500시간 작동에도 성능이 감소하지 않는 것을 확인했으며, 800oC에서 수소/공기 공급시 최대 성능 1.61W/cm2를 보여 BCFZY와 함께 고성능, 고내구성을 가진 또 다른 TCO라 할 수 있다.48
3. 기타 환원극
계층화 구조를 가진 LNO (LiNiO2)를 주 물질 구조로 하나 여기에 Co를 도핑하여 SOFC용 TCO 환원극으로 이용한 사례가 있다. 계층화된 LNO의 특성상 Co를 도핑하여 LNCO (LiNi0.8Co0.2O2)를 합성하면 Ni가 부분적으로 Co로 치환되어 니켈의 리튬이온층으로의 이동을 막게되어 계층 구조를 더욱 강화하게 되는 효과가 있다.49 여기에 LNO 자체의 ORR 반응성과 도핑에 의해 인위적으로 만들어진 수소이온전도성이 만나 SOFC용 삼중 전도성 환원극으로서의 높은 가능성을 보여준다. 본 연구에서 최종적으로 Ni-BCZY (BaCe0.8Zr0.1Y0.1O3-δ) |BCZY|LNCO-BCZY 구조의 SOFC에서 수소연료 이용시 650oC에서 최대전력밀도 410 mW/cm2의 성능을 보이는 것을 확인했다. 이밖에 러들스든-포퍼(Ruddlesden-Popper) 구조의 Pr2NiO3-δ 형태의 물질이 삼중 전도성을 보여 SOFC 환원극으로서의 가능성을 보여준다는 보고가 있으나 대칭 셀 구성을 통한 전기화학적 임피던스 분석을 통한 결과 외에 SOFC용 환원극으로 직접 사용된 사례는 없는 것으로 파악된다.50
4. 결론
본 논문에서는 TCO로 알려진 여러 물질들을 원자 구조에 따라 페로브스카이트, 계층화된 페로브스카이트, 그리고 이 외 기타로 간단히 나눠보았다. 본문의 내용과 같이 TCO의 SOFC용 환원극으로의 가능성이 실험적으로 제안은 되었으나, 아직 물질의 장기 안정성, 대량 생산 등의 상용화 관련 기술 논의는 초기단계에 가깝다. 특히 본문에서 재조명된 여러 신 TCO 전극 합성은 아직 솔-겔 방법을 위주로 하여, 향후 저비용 및 대량생산이 용이한 방법이 개발될 필요가 있으며, TCO의 환원극로의 적용 방법에 관한 연구도 필요하다. 그러나 본 논문에서 재조명된 TCO의 환원극으로서의 실험적 결과는 기존 문헌상 최대 전기화학적 성능을 보이는 타 환원극의 성능에 필적하는, 또는 이를 상회하는 수준의 성능을 보인다. 특히 보고된 물질의 양 대비 TCO가 보이는 SOFC용 환원극으로서의 ORR 활성도 및 내구성은 매우 탁월하여, 향후 차세대 SOFC의 주 물질로 사용될 가능성이 높은 것으로 판단된다.
Acknowledgments
본 연구는 2018년도 숭실대학교 교내연구비 지원에 의한 연구임.
REFERENCES
-
Beckel, D., Bieberle-Hütter, A., Harvey, A., Infortuna, A., Muecke, U. P., et al., “Thin Films for Micro Solid Oxide Fuel Cells,” Journal of Power Sources, Vol. 173, No. 1, pp. 325-345, 2007.
[https://doi.org/10.1016/j.jpowsour.2007.04.070]
-
Jacobson, A. J., “Materials for Solid Oxide Fuel Cells,” Chem. Mater, Vol. 22, No. 3, pp. 660-674, 2010.
[https://doi.org/10.1021/cm902640j]
-
Wilberforce, T., Alaswad, A., Palumbo, A., Dassisti, M., and Olabi, A., “Advances in Stationary and Portable Fuel Cell Applications,” International Journal of Hydrogen Energy, Vol. 41, No. 37, pp. 16509-16522, 2016.
[https://doi.org/10.1016/j.ijhydene.2016.02.057]
-
Alaswad, A., Baroutaji, A., Achour, H., Carton, J., Al Makky, A., et al., “Developments in Fuel Cell Technologies in the Transport Sector,” International Journal of Hydrogen Energy, Vol. 41, No. 37, pp. 16499-16508, 2016.
[https://doi.org/10.1016/j.ijhydene.2016.03.164]
-
O'hayre, R., Cha, S.-W., Prinz, F. B., and Colella, W., “Fuel Cell Fundamentals,” John Wiley & Sons, 2016.
[https://doi.org/10.1002/9781119191766]
-
Ormerod, R. M., “Solid Oxide Fuel Cells,” Chemical Society Reviews, Vol. 32, No. 1, pp. 17-28, 2003.
[https://doi.org/10.1039/b105764m]
-
Badwal, S. and Foger, K., “Solid Oxide Electrolyte Fuel Cell Review,” Ceramics International, Vol. 22, No. 3, pp. 257-265, 1996.
[https://doi.org/10.1016/0272-8842(95)00101-8]
-
Stambouli, A. B. and Traversa, E., “Solid Oxide Fuel Cells (SOFCs): A Review of an Environmentally Clean and Efficient Source of Energy,” Renewable and Sustainable Energy Reviews, Vol. 6, No. 5, pp. 433-455, 2002.
[https://doi.org/10.1016/S1364-0321(02)00014-X]
-
Lee, Y. H., Chang, I., Cho, G. Y., Park, J., Yu, W., et al., “Thin Film Solid Oxide Fuel Cells Operating Below 600° c: A Review,” International Journal of Precision Engineering and Manufacturing-Green Technology, Vol. 5, No. 3, pp. 441-453, 2018.
[https://doi.org/10.1007/s40684-018-0047-0]
-
Kim, Y., Noh, S., Cho, G. Y., Park, T., Lee, Y. H., et al., “Characterization of Thin Film Solid Oxide Fuel Cells with Variations in the Thickness of Nickel Oxide-Gadolinia Doped Ceria Anode,” International Journal of Precision Engineering and Manufacturing, Vol. 17, No. 8, pp. 1079-1083, 2016.
[https://doi.org/10.1007/s12541-016-0131-8]
-
Kerman, K., Lai, B.-K., and Ramanathan, S., “Pt/y0.16Zr0.84O1.92/Pt Thin Film Solid Oxide Fuel Cells: Electrode Microstructure and Stability Considerations,” Journal of Power Sources, Vol. 196, No. 5, pp. 2608-2614, 2011.
[https://doi.org/10.1016/j.jpowsour.2010.10.068]
-
Jiang, S., Yi, B., Cao, L., Song, W., Zhao, Q., et al., “Development of Advanced Catalytic Layer Based on Vertically Aligned Conductive Polymer Arrays for Thin-Film Fuel Cell Electrodes,” Journal of Power Sources, Vol. 329, No. pp. 347-354, 2016.
[https://doi.org/10.1016/j.jpowsour.2016.08.098]
-
Park, J., Lee, Y., Chang, I., Lee, W., and Cha, S. W., “Engineering of the Electrode Structure of Thin Film Solid Oxide Fuel Cells,” Thin Solid Films, Vol. 584, No. pp. 125-129, 2015.
[https://doi.org/10.1016/j.tsf.2014.11.018]
-
Su, P.-C., Chao, C.-C., Shim, J. H., Fasching, R., and Prinz, F. B., “Solid Oxide Fuel Cell with Corrugated Thin Film Electrolyte,” Nano Letters, Vol. 8, No. 8, pp. 2289-2292, 2008.
[https://doi.org/10.1021/nl800977z]
-
An, J., Kim, Y.-B., Park, J., Gür, T. M., and Prinz, F. B., “Three-Dimensional Nanostructured Bilayer Solid Oxide Fuel Cell With 1.3 W/cm2 at 450oC,” Nano Letters, Vol. 13, No. 9, pp. 4551-4555, 2013.
[https://doi.org/10.1021/nl402661p]
-
Hong, S., Bae, J., Koo, B., and Kim, Y.-B., “High-Performance Ultra-Thin Film Solid Oxide Fuel Cell Using Anodized-Aluminum-Oxide Supporting Structure,” Electrochemistry Communications, Vol. 47, No. pp. 1-4, 2014.
[https://doi.org/10.1016/j.elecom.2014.07.008]
-
Yu, W., Lee, Y., Lee, Y. H., Cho, G. Y., Park, T., et al., “Performance Enhancement of Thin Film LSCF Cathodes by Gold Current Collecting Layer,” International Journal of Precision Engineering and Manufacturing-Green Technology, Vol. 3, No. 2, pp. 185-188, 2016.
[https://doi.org/10.1007/s40684-016-0024-4]
-
Park, T., Cho, G. Y., Lee, Y. H., Tanveer, W. H., Yu, W., et al., “Effect of Anode Morphology on the Performance of Thin Film Solid Oxide Fuel Cell with Peald YSZ Electrolyte,” International Journal of Hydrogen Energy, Vol. 41, No. 22, pp. 9638-9643, 2016.
[https://doi.org/10.1016/j.ijhydene.2016.04.092]
-
Chang, I.-W., Ji, S.-H., Paek, J.-Y., Lee, Y.-H., Park, T.-H., et al., “Study on Low-Temperature Solid Oxide Fuel Cells Using Y-Doped BaZrO3,” Transactions of the Korean Society of Mechanical Engineers B, Vol. 36, No. 9, pp. 931-935, 2012.
[https://doi.org/10.3795/KSME-B.2012.36.9.931]
-
Park, T., Cho, G. Y., Lee, Y. H., Tanveer, W. H., Yu, W., et al., “Effect of Anode Morphology on the Performance of Thin Film Solid Oxide Fuel Cell with Peald YSZ Electrolyte,” International Journal of Hydrogen Energy, Vol. 41, No. 22, pp. 9638-9643, 2016.
[https://doi.org/10.1016/j.ijhydene.2016.04.092]
-
Shim, J. H., Kang, S., Cha, S.-W., Lee, W., Kim, Y. B., et al., “Atomic Layer Deposition of Thin-Film Ceramic Electrolytes for High-Performance Fuel Cells,” Journal of Materials Chemistry A, Vol. 1, No. 41, pp. 12695-12705, 2013.
[https://doi.org/10.1039/c3ta11399j]
-
Ignatiev, A., Chen, X., Wu, N., Lu, Z., and Smith, L., “Nanostructured Thin Solid Oxide Fuel Cells with High Power Density,” Dalton Transactions, Vol., No. 40, pp. 5501-5506, 2008.
[https://doi.org/10.1039/b805658g]
-
Ji, S., Cho, G. Y., Yu, W., Su, P.-C., Lee, M. H., et al., “Plasma-Enhanced Atomic Layer Deposition of Nanoscale Yttria-Stabilized Zirconia Electrolyte for Solid Oxide Fuel Cells with Porous Substrate,” ACS Applied Materials & Interfaces, Vol. 7, No. 5, pp. 2998-3002, 2015.
[https://doi.org/10.1021/am508710s]
-
Kang, S., Heo, P., Lee, Y. H., Ha, J., Chang, I., et al., “Low Intermediate Temperature Ceramic Fuel Cell with Y-Doped BaZrO3 Electrolyte and Thin Film PD Anode on Porous Substrate,” Electrochemistry Communications, Vol. 13, No. 4, pp. 374-377, 2011.
[https://doi.org/10.1016/j.elecom.2011.01.029]
-
Park, T., Lee, Y. H., Cho, G. Y., Ji, S., Park, J., et al., “Effect of the Thickness of Sputtered Gadolinia-Doped Ceria as a Cathodic Interlayer in Solid Oxide Fuel Cells,” Thin Solid Films, Vol. 584, pp. 120-124, 2015.
[https://doi.org/10.1016/j.tsf.2015.03.010]
-
Park, T., Chang, I., and Cha, S. W., “Augmentation Method of Triple Phase Boundary in Thin Film Solid Oxide Fuel Cell Via Physical Vapor Deposition,” Journal of Nanoscience and Nanotechnology, Vol. 13, No. 12, pp. 7834-7838, 2013.
[https://doi.org/10.1166/jnn.2013.8102]
-
Cha, S. W., Cho, G. Y., Lee, Y., Park, T., Kim, Y., et al., “Effects of Carbon Contaminations on Y2O3-Stabilized ZrO2 Thin Film Electrolyte Prepared by Atomic Layer Deposition for Thin Film Solid Oxide Fuel Cells,” CIRP Annals, Vol. 65, No. 1, pp. 515-518, 2016.
[https://doi.org/10.1016/j.cirp.2016.04.079]
-
Ha, S., Su, P.-C., and Cha, S.-W., “Combinatorial Deposition of a Dense Nano-Thin Film YSZ Electrolyte for Low Temperature Solid Oxide Fuel Cells,” Journal of Materials Chemistry A, Vol. 1, No. 34, pp. 9645-9649, 2013.
[https://doi.org/10.1039/c3ta11758h]
-
Chang, I., Bae, J., Park, J., Lee, S., Ban, M., et al., “A Thermally Self-Sustaining Solid Oxide Fuel Cell System at Ultra-Low Operating Temperature (319°C),” Energy, Vol. 104, pp. 107-113, 2016.
[https://doi.org/10.1016/j.energy.2016.03.099]
-
Shim, J. H., Chao, C.-C., Huang, H., and Prinz, F. B., “Atomic Layer Deposition of Yttria-Stabilized Zirconia for Solid Oxide Fuel Cells,” Chemistry of Materials, Vol. 19, No. 15, pp. 3850-3854, 2007.
[https://doi.org/10.1021/cm070913t]
-
Ji, S., Ha, J., Park, T., Kim, Y., Koo, B., et al., “Substrate-Dependent Growth of Nanothin Film Solid Oxide Fuel Cells Toward Cost-Effective Nanostructuring,” International Journal of Precision Engineering and Manufacturing-Green Technology, Vol. 3, No. 1, pp. 35-39, 2016.
[https://doi.org/10.1007/s40684-016-0005-7]
-
Huang, H., Nakamura, M., Su, P., Fasching, R., Saito, Y., et al., “High-Performance Ultrathin Solid Oxide Fuel Cells for Low-Temperature Operation,” Journal of the Electrochemical Society, Vol. 154, No. 1, pp. 20-24, 2007.
[https://doi.org/10.1149/1.2372592]
-
Park, J., Chang, I., Paek, J. Y., Ji, S., Lee, W., et al., “Fabrication of the Large Area Thin-Film Solid Oxide Fuel Cells,” CIRP Annals-Manufacturing Technology, Vol. 63, No. 1, pp. 513-516, 2014.
[https://doi.org/10.1016/j.cirp.2014.03.065]
-
Park, T., Lee, Y. H., Cho, G. Y., Ji, S., Park, J., et al., “Effect of the Thickness of Sputtered Gadolinia-Doped Ceria as a Cathodic Interlayer in Solid Oxide Fuel Cells,” Thin Solid Films, Vol. 584, pp. 120-124, 2015.
[https://doi.org/10.1016/j.tsf.2015.03.010]
-
Chang, I., Woo, S., Lee, M. H., Shim, J. H., Piao, Y., et al., “Characterization of Porous pt Films Deposited Via Sputtering,” Applied Surface Science, Vol. 282, pp. 463-466, 2013.
[https://doi.org/10.1016/j.apsusc.2013.05.153]
-
An, J., Kim, Y. B., Jung, H. J., Park, J. S., Cha, S. W., et al., “Structural and Compositional Analysis of Solid Oxide Fuel Cell Electrolytes Using Transmission Electron Microscopy,” International Journal of Precision Engineering and Manufacturing, Vol. 13, No. 7, pp. 1273-1279, 2012.
[https://doi.org/10.1007/s12541-012-0170-8]
-
Fabbri, E., Pergolesi, D., and Traversa, E., “Materials Challenges Toward Proton-Conducting Oxide Fuel Cells: A Critical Review,” Chemical Society Reviews, Vol. 39, No. 11, pp. 4355-4369, 2010.
[https://doi.org/10.1039/b902343g]
-
Tanveer, W. H., Ji, S., Yu, W., Cho, G. Y., Lee, Y. H., et al., “Effect of 20% O2 Reactive Gas on RF-Sputtered Ni-SDC Cermet Anodes for Intermediate Temperature Solid Oxide Fuel Cells,” Current Applied Physics, Vol. 16, No. 12, pp. 1680-1686, 2016.
[https://doi.org/10.1016/j.cap.2016.10.003]
- Kharton, V., Figueiredo, F., Navarro, L., Naumovich, E., Kovalevsky, A., et al, “Ceria-Based Materials for Solid Oxide Fuel Cells,” Journal of Materials Science, Vol. 36, No. 5, pp. 1105-1117, 2001.
-
Tsoga, A., Gupta, A., Naoumidis, A., and Nikolopoulos, P., “Gadolinia-Doped Ceria and Yttria Stabilized Zirconia Interfaces: Regarding Their Application for SOFC Technology,” Acta Materialia, Vol. 48, No. 18-19, pp. 4709-4714, 2000.
[https://doi.org/10.1016/S1359-6454(00)00261-5]
-
Shao, Z. and Haile, S.M., “A High-Performance Cathode for the Next Generation of Solid-Oxide Fuel Cells,” Nature, Vol. 431, No. 7005, p. 170, 2004.
[https://doi.org/10.1038/nature02863]
-
Leng, Y., Chan, S. H., and Liu, Q., “Development of LSCF–GDC Composite Cathodes for Low-Temperature Solid Oxide Fuel Cells with Thin Film GDC Electrolyte,” International Journal of Hydrogen Energy, Vol. 33, No. 14, pp. 3808-3817, 2008.
[https://doi.org/10.1016/j.ijhydene.2008.04.034]
-
Norby, T., “Solid-State Protonic Conductors: Principles, Properties, Progress and Prospects,” Solid State Ionics, Vol. 125, Nos. 1-4, pp. 1-11, 1999.
[https://doi.org/10.1016/S0167-2738(99)00152-6]
-
Serra, J. M. and Meulenberg, W. A., “Thin?Film Proton BaZr0.85Y0.15O3 Conducting Electrolytes: Toward an Intermediate Temperature Solid Oxide Fuel Cell Alternative,” Journal of the American Ceramic Society, Vol. 90, No. 7, pp. 2082-2089, 2007.
[https://doi.org/10.1111/j.1551-2916.2007.01677.x]
-
Fabbri, E., Pergolesi, D., and Traversa, E., “Materials Challenges Toward Proton-Conducting Oxide Fuel Cells: A Critical Review,” Chemical Society Reviews, Vol. 39, No. 11, pp. 4355-4369, 2010.
[https://doi.org/10.1039/b902343g]
-
Ni, M., “The Effect of Electrolyte Type on Performance of Solid Oxide Fuel Cells Running on Hydrocarbon Fuels,” International Journal of Hydrogen Energy, Vol. 38, No. 6, pp. 2846-2858, 2013.
[https://doi.org/10.1016/j.ijhydene.2012.12.055]
-
Duan, C., Tong, J., Shang, M., Nikodemski, S., Sanders, M., et al., “Readily Processed Protonic Ceramic Fuel Cells with High Performance at Low Temperatures,” Science, Vol. 349, No. 6254, pp. 1321-1326, 2015.
[https://doi.org/10.1126/science.aab3987]
-
Kim, J., Sengodan, S., Kwon, G., Ding, D., Shin, J., et al., “Triple Conducting Layered Perovskites as Cathode Materials for Proton Conducting Solid Oxide Fuel Cells,” ChemSusChem, Vol. 7, No. 10, pp. 2811-2815, 2014.
[https://doi.org/10.1002/cssc.201402351]
-
Fan, L. and Su, P.-C., “Layer-structured LiNi0.8Co0.2O2 : A New Triple (H+/O2-/e-) Conducting Cathode for Low Temperature Proton Conducting Solid Oxide Fuel Cells,” Journal of Power Sources, Vol. 306, pp. 369-377, 2016.
[https://doi.org/10.1016/j.jpowsour.2015.12.015]
-
Grimaud, A., Mauvy, F., Bassat, J., Fourcade, S., Rocheron, L., et al., “Hydration Properties and Rate Determining Steps of the Oxygen Reduction Reaction of Perovskite-Related Oxides as H+-SOFC Cathodes,” Journal of The Electrochemical Society, Vol. 159, No. 6, pp. 683-694, 2012.
[https://doi.org/10.1149/2.101205jes]
-
Duan, C., Hook, D., Chen, Y., Tong, J., and O'Hayre, R., “Zr and Y Co-Doped Perovskite as a Stable, High Performance Cathode for Solid Oxide Fuel Cells Operating Below 500oC,” Energy & Environmental Science, Vol. 10, No. 1, pp. 176-182, 2017.
[https://doi.org/10.1039/C6EE01915C]
-
Shang, M., Tong, J., and O'Hayre, R., “A Promising Cathode for Intermediate Temperature Protonic Ceramic Fuel Cells: BaCo0.4Fe0.4Zr0.2O3-δ,” RSC Advances, Vol. 3, No. 36, pp. 15769-15775, 2013.
[https://doi.org/10.1039/c3ra41828f]
-
Tong, J., Yang, W., Zhu, B., and Cai, R., “Investigation of Ideal Zirconium-Doped Perovskite-Type Ceramic Membrane Materials for Oxygen Separation,” Journal of Membrane Science, Vol. 203, Nos. 1-2, pp. 175-189, 2002.
[https://doi.org/10.1016/S0376-7388(02)00005-4]
-
Choi, S., Yoo, S., Kim, J., Park, S., Jun, A., et al., “Highly Efficient and Robust Cathode Materials for Low-Temperature Solid Oxide Fuel Cells: PrBa0.5Sr0.5Co2−xFexO5+δ,” Scientific Reports, Vol. 3, p. 2426, 2013.
[https://doi.org/10.1038/srep02426]
-
Kim, G., Wang, S., Jacobson, A., Reimus, L., Brodersen, P., et al., “Rapid Oxygen Ion Diffusion and Surface Exchange Kinetics in PrBaCo2O5+x with a perovskite related structure and ordered A cations,” Journal of Materials Chemistry, Vol. 17, No. 24, pp. 2500-2505, 2007.
[https://doi.org/10.1039/b618345j]
-
Tarancón, A., Skinner, S. J., Chater, R. J., Hernandez-Ramirez, F., and Kilner, J. A., “Layered Perovskites as Promising Cathodes for Intermediate Temperature Solid Oxide Fuel Cells,” Journal of Materials Chemistry, Vol. 17, No. 30, pp. 3175-3181, 2007.
[https://doi.org/10.1039/b704320a]
-
Taskin, A., Lavrov, A., and Ando, Y., “Achieving Fast Oxygen Diffusion in Perovskites by Cation Ordering,” Applied Physics Letters, Vol. 86, No. 9, Paper No. 091910, 2005.
[https://doi.org/10.1063/1.1864244]
-
Jun, A., Shin, J., and Kim, G., “High Redox and Performance Stability of Layered SmBa0.5Sr0.5Co1.5Cu0.5O5+δ perovskite cathodes for intermediate-temperature solid oxide fuel cells,” Physical Chemistry Chemical Physics, Vol. 15, No. 45, pp. 19906-19912, 2013.
[https://doi.org/10.1039/c3cp53883d]
-
Yoo, S., Choi, S., Kim, J., Shin, J., and Kim, G., “Investigation of Layered Perovskite Type NdBa1−xSrxCo2O5+δ (x=0, 0.25, 0.5, 0.75, and 1.0) cathodes for intermediate-temperature solid oxide fuel cells,” Electrochimica Acta, Vol. 100, pp. 44-50, 2013.
[https://doi.org/10.1016/j.electacta.2013.03.041]
Assistant Professor, School of Mechanical Engineering, Soongsil University. Research Interest: Fuel Cells
E-mail: taehyunpark@ssu.ac.kr