
척추부하 평가를 위한 L4-L5 요추의 유한요소모델 개발
Copyright © The Korean Society for Precision Engineering
This is an Open-Access article distributed under the terms of the Creative Commons Attribution Non-Commercial License (http://creativecommons.org/licenses/by-nc/3.0) which permits unrestricted non-commercial use, distribution, and reproduction in any medium, provided the original work is properly cited.
Abstract
Low back injury (LBI) often occurs during manual materials handling (MMH). Intradiscal pressure (IDP) is used to assess the risk of the LBI and is measured in vivo or by computer simulation. As for computer simulation, motion analysis and finite element (FE) analysis are usually employed. In this study, a FE model has been developed for L4-L5 segment with high risk of injury to predict LBI during manual lifting tasks. The FE model was composed of lumbar vertebrae, discs, and ligaments and a calibration process was performed to set the nonlinear material properties of the intervertebral disc. To validate the developed FE model, IDP and range of motion (ROM) under in vitro loading conditions were compared to the experiments and other FE studies in literature. Within in vitro range, IDP and ROM from the FE model were in agreement with results from previous studies. The FE model developed in this study can be scaled according to the subject used in the analysis integrating FE analysis to motion analysis, and is expected to be used in future work to estimate IDP and stress/strain in joint structures during occupational activities.
Keywords:
Lumbar spine, Finite element model, Intradiscal pressure, Range of motion키워드:
요추, 유한요소모델, 추간판 내부압력, 운동 가동 범위1. 서론
요추 질환(Low Back Pain)은 모든 연령에 있어 가장 흔하게 발생하며 장애의 위험이 큰 질병 중 하나다.1 미국의 경우 연간 1,000억 달러 이상의 높은 진단 비용이 발생하며, 성인 인구의 약 2/3가 요통을 경험한다. 요통은 24-80%의 높은 재발률을 지니며, 2015년 보고된 전 세계 활동 제한 유형의 요통은 7.3%로 5억 4천만명에 이른다.2,3
중량물 취급작업(MMH)은 척추에 상당한 부하를 발생시키고, 특히 굽힘 자세에서 무거운 중량물을 반복적으로 다루는 작업은 요추 부상을 일으키는 주요 원인이다.4,5 이는 추간판 내부압력(IDP) 증가, 요추 염좌, 종판 파단, 추간판 탈출증과 같은 질환으로 이어질 수 있는 위험이 존재한다. 중량물 취급 작업 시 L4-L5, L5-S 분절은 요추에서 가장 큰 압축력과 모멘트가 발생하며, 추간판은 이로 인한 부상의 위험이 가장 큰 조직이다.6,7 이러한 요추 부상의 위험을 예측하기 위한 방법으로 추간판 내부압력이 일반적으로 사용된다. 추간판 내부압력을 구하는 방법에는 생체 실험을 통해 측정하는 방법과 컴퓨터 시뮬레이션에 의해 얻는 해석적 방법이 있다.
생체 내 측정 방법(In vivo)은 침습적인 장치로 추간판 내부압력을 측정하는 방법이다.8-10 많은 비용과 기술적·윤리적 제약이 따르며, 다양한 환경 및 피험자에 대한 실험이나 추간판(Disc), 섬유륜(Annulus Fibrosus), 종판(Endplate), 인대(Ligament) 등의 내부 조직이 받는 영향에 대한 분석이 어렵다. 생체 내 측정 방법(In vivo)의 한계를 보완하기 위해 활용되는 해석적 방법에는 두 가지가 있는데, 동작분석(Motion Analysis)에 의해 동적 상태에서 구하는 방법과 유한요소법을 활용한 정적 상태에서 구하는 방법이 있다.
동작분석은 강체 동역학(Rigid Body Dynamics) 기반의 인체 모델을 활용하여 동작에 따라 척추 분절의 운동과 주변 근육 힘을 추정한다.7,11 추간판 내부압력을 계산하기 위해 척추 관절에 작용하는 압축력과 회전 변위에 대한 경험식을 활용하며 일정한 크기의 추간판 단면적을 가정한다.12,13 이와 같은 강체 모델을 활용한 해석은 추간판 L4-L5 분절의 굽힘(Flexion) 각도에 대한 경험식을 활용하기 때문에 굽힘 자세 이외의 복잡한 동작에 따른 추간판 내부압력을 측정하기 어려운 한계가 있다. 반면 유한요소해석은 추간판의 복잡한 구조와 비선형 물성을 고려한 유한요소모델(Finite Element Model)을 활용하여 추간판의 기계적 거동에 대한 분석이 용이하다. 요추 만곡에 따라 일정한 종동력(Follower Load)을 부과하는 생체 외 측정(In vitro) 연구의 실험적 방법을 적용하여 척추 주변 근육 힘을 보완한다.14,15 이는 생체 실험법에 근거하는 하중 조건으로 굽힘(Flexion), 신전(Extension), 측굴(Lateral Bending), 축회전(Axial Rotation)과 같은 기본적인 척추 거동 이외의 동작에 대한 분석이 어렵고, 주변 근육의 능동적인 힘을 모사하지 못하는 한계를 지닌다.
최근에는 동작 분석과 유한요소해석을 연계하여 보행 시 하체의 무릎 연골 및 발목 관절의 내부응력을 분석하는 연구를 수행하였다.16,17 이러한 해석기법의 연계는 각 방법의 한계점을 보완하는 동시에 강점을 고려하여 동작 중인 인체에 대한 해석을 가능하게 한다. 선행연구에서 활용된 방법을 상체 모델에 확장하여 적용하면 작업 동작 중에 발생하는 추간판의 내부응력과 변형 등을 분석할 수 있다. 본 연구에서는 동작 분석과 연계하여 작업 동작 중 요추의 생체역학적 변화를 측정하기 위한 상세 유한요소모델을 개발하고자 하였다. 지금까지 다수의 연구에서 요추부 유한요소모델 개발과 In vitro 실험 결과와 비교를 통한 모델의 검증이 수행되었다. Xu, et al., Mills, et al.은 1,000 N의 순수 압축힘, 10 N·m의 순수 모멘트, 압축힘과 모멘트를 동시에 인가하는 복합 하중(Combined Loading)을 적용하여 In vitro 실험연구와 동일한 하중 조건에서 유한요소모델을 검증하였다.18,19 이러한 연구는 척추 운동에 따른 단순하중 및 복합 하중(Combined Loading)을 고려하여 모델의 타당성을 검증하였으나, 영역에 따른 추간판의 비선형적 거동 특성을 상세히 고려하지 않은 한계가 있다. Schmidt, et al.은 Calibration Method를 개발하고, 추간판 내부 섬유륜의 층과 방향에 따른 가중치를 적용하여 비선형 물성을 구현하였으나, 순수 모멘트의 단순 하중 조건에서만 모델 검증이 이루어졌다.20 이러한 기존 연구를 보완하여 본 연구에서는 이상적인 추간판 물성을 구현한 요추부 표준 유한요소모델을 개발하고, 척추 거동에 대한 하중 조건을 고려하여 모델을 검증하였다.
본 연구에서는 피험자의 신장, 체중 등의 신체 정보를 반영하여 동작 분석과 유한요소해석의 연계에 활용 가능한 요추부 표준 유한요소모델을 개발하였다. 본 연구의 목적은 척추 추간판의 기저층, 섬유륜, 종판과 주변 인대의 상세 모델링을 통해 요추 제4-5번(L4-L5)의 유한요소모델을 개발하고, 요추부 표준 유한요소모델에 가장 이상적인 물성을 적용하여 기존의 생체 실험 연구 및 유한요소 연구 결과와 비교하여 모델 검증을 수행하는 것이다.
2. 유한요소모델
2.1 요추부 형상 모델
본 연구에서 개발된 요추 유한요소모델의 형상은 Visible Human Project에서 제공하는 MRI의 3차원 인체 형상 데이터를 활용하였다.21 추간판의 크기는 미국 성인 남성의 표준 데이터와 기존 측정 문헌을 참고하였으며,22-24 상용프로그램인 Rapidform XOR3 64 (3D Systems Inc., USA)를 이용하여 모델링하였다. 제4-5번 요추의 전만각(Lordosis Angle)은 12.2o이며, 문헌을 참고하여 무부하 상태를 반영하는 누움 자세의 각도를 적용하였다.25
2.2 요추부 유한요소모델
요추부 유한요소모델은 요추 제4-5번을 대상으로 요추체(L4-L5), 추간판, 인대(Ligament)로 구성하였으며 상용 프로그램(Hypermesh 13.0, Altair Engineering Inc., USA)을 활용하여 요소망을 생성하였다. 추체의 경계에는 1 mm로 일정한 두께의 종판이 골성 종판(Bony Endplate)과 연골성 종판(Cartilaginous Endplate)으로 이루어지며, 추간판은 중심부에 위치한 수핵(Nucleus Pulposus)과 이를 둘러싸는 섬유륜으로 구성한다(Fig. 1). 섬유륜은 여러 층의 기저층(Ground Substance)과 그 사이에 8겹의 섬유소(Annulus Fiber)가 내재되어 있는 구조로 수평축에 대해 평균 35o로 기울어져 교차하는 형태이며,26 이상적인 섬유소 물성을 적용하기 위해 Schmidt, et al.의 Calibration Method를 활용하여 4가지 층과 5가지 방향의 총 20가지 영역에 따라 다른 물성을 적용하였다(Fig. 2).20,27
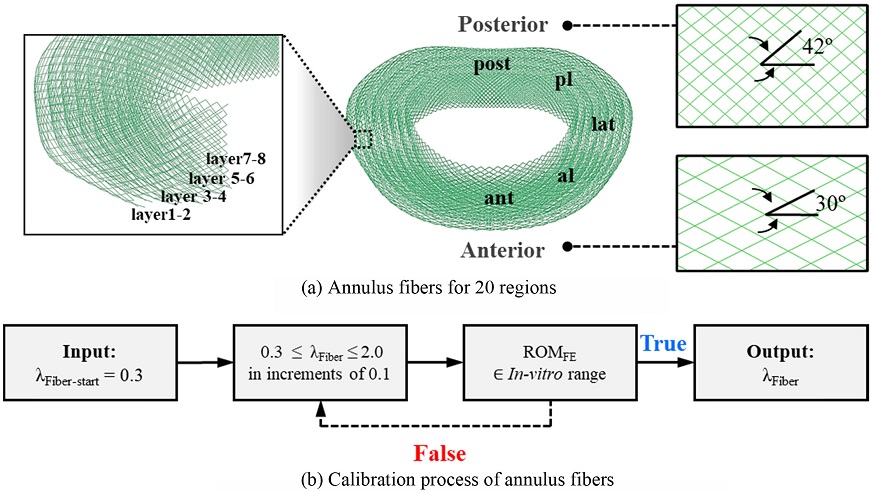
Detailed views of annulus fiber (ant: Anterior, al: Antero-Lateral, lat: Lateral, pl: Postero-Lateral, post: Posterior)
본 연구에서는 유한요소해석 프로그램으로 ABAQUS ver.6.14(Dassault Systems Simulia Corp., USA)를 사용하였다. 추간판 내부의 수핵은 정수압 거동을 구현하는 4절점 유체 요소를 활용하였으며, 수분과 동일한 체적 탄성률(Bulk Modulus)과 초기압력(Initial Pressure)을 적용하였다. 섬유륜 기저층은 비압축성 성질의 솔리드 요소를 활용하여 초탄성 특성의 무니-리블린 모델을 적용하였으며, 섬유소는 인장에 대한 저항만 가지는 2절점 요소로 모델링하였다.
섬유소의 기계적 특성은 응력-변형률 곡선에 따른 비선형 거동을 따르도록 구현하였으며, 영역에 따른 가중치를 적용하여 가장 바깥층의 섬유소가 가장 강한 강성을 지니도록 하였다(Outermost Layers 1-2: 1.0, Layers 3-4: 0.9, Layers 5-6: 0.75, Innermost Layers 7-8: 0.65).27 강체인 추골은 4절점 솔리드와 5절점 솔리드 요소를 혼합하여 구성하였으며, 요추 모델에 사용된 물성치는 기존 문헌을 참조하였다(Table 1).20,28-30
척추를 연결하는 인대는 해부학적 문헌을 참고하여 7개의 주요 인대(Anterior Longitudinal Ligament, ALL; Posterior Longitudinal Ligament, PLL; Supraspinous Ligament, SSL; Interspinous Ligament, ISL; Intertransverse Ligament, ITL; Ligamentum Flavum, LF; Capsular Ligament, CL)를 구현하였으며, 인장에 대한 저항을 가지는 단축(Uni-Axial) 구조의 2절점 요소로 비선형 탄성 거동을 구현하였다.31
2.3 유한요소모델 검증
본 연구에서 개발된 요추 제4-5번(L4-L5) 상세 유한요소모델에 대한 검증을 위하여 해석을 통해 수핵의 중심부에서 도출된 추간판 내부압력과 운동 가동 범위(ROM) 결과를 기존의 In vitro 실험 및 타 유한요소 연구 결과와 비교하여 분석하였다.8,9,32-38
하중 조건은 대표적으로 검증에 활용되는 순수 압축 하중, 4가지 방향의 순수 모멘트, 복합 하중을 적용하였다(Fig. 1). 요추 제5번을 모든 축에 대해 구속한 상태에서 순수 압축 하중 1,000 N을 가하여 하중 시 증가하는 추간판 내부압력를 분석하였으며, 4가지 방향(굽힘, 신전, 측굴, 축회전)에 대해 10 N·m의 순수 모멘트를 가하여 척추 분절의 운동 가동 범위(ROM)를 측정하였다(Table 2).36-38
3. 검증 결과
3.1 순수 압축 하중
요추 제4-5번 모델의 검증을 위하여 순수 압축 하중(1,000 N)에 따른 추간판 내부압력 변화를 In vitro 실험 및 타 유한요소연구 결과와 비교·검증하였다.8,31,34,38
추간판 내부압력은 In vitro 실험 및 기존 유한요소연구의 결과 범위 내에서 압축 하중에 비례하여 선형적으로 증가하는 경향을 보였으며, 기존 문헌들과 유사한 결과를 나타내며 1,000 N의 압축 하중이 인가되었을 때 In vitro 실험 결과와 11.2%의 오차를 나타냈다(Fig. 3).
3.2 순수 모멘트
단분절 요추 모델에 대해 굴곡/신전/측굴/축회전의 순수 모멘트(10 N·m)를 가하여 척추 분절의 운동 가동 범위(ROM)를 측정하였다. 본 연구에서 개발한 요추 모델은 Heuer 사체 실험 결과의 범위 내에 존재함을 확인하였으며, 기존 유한요소 연구 결과와 유사한 경향을 나타냈다(Fig. 4).9,32,34,35
3.3 복합 하중
복합 하중은 척추 운동과 가장 근접한 하중 조건으로 근육의 결손을 대체하는 종동력과 회전 모멘트를 부과하여 추간판 내부압력과 분절의 운동 가동 범위를 측정하였다. In vivo Median은 문헌으로부터 Flexion/Extension, Lateral Bending, Axial Rotation에서 각각 11명, 10명, 10명의 피험자에 대해 측정된 값을 사용하였다.39
모든 결과는 문헌의 결과 범위 내에 존재하였으며, 유한요소 연구 결과의 중앙값과 가장 일치하는 값을 보였다(Figs. 5와 6).33,36,37
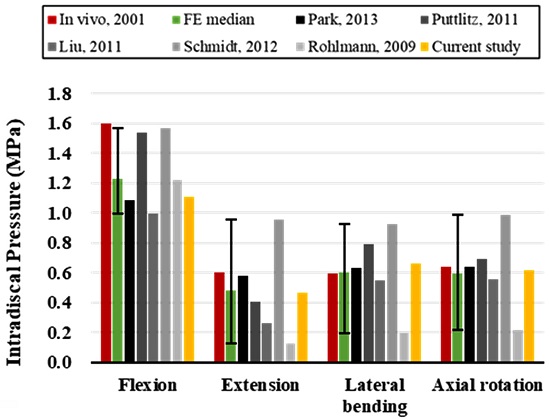
Comparison between predicted intradiscal pressure (IDP) of present FE model and the existing in vivo measurement and FE models under combined loading
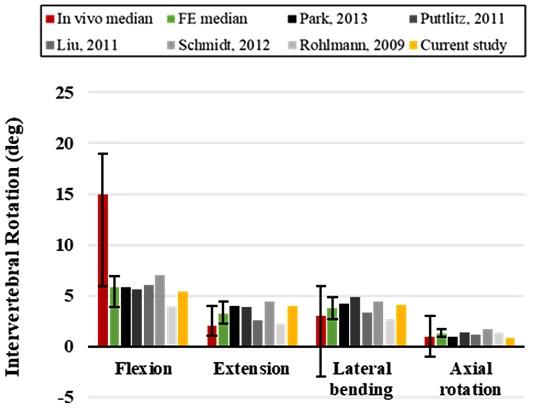
Comparison between range of motion (ROM) of present FE model and the existing in vivo measurement and FE models under combined loading
In vivo 실험 결과와 비교하였을 때 추간판 내부압력은 기존의 유한요소 연구와 유사하게 굽힘 및 신전에서 다소 낮은 값을 보였으나 측굴과 축회전에서 높은 일치율을 나타냈다. 운동 가동 범위 결과는 굽힘에서 생체 실험보다 낮은 값을 보였으나 하중 조건에 따른 경향은 생체 실험 결과와 유사하게 나타났다.
4. 결론
본 연구에서는 작업 자세에 따른 요추의 생체역학적 분석을 위해 동작분석과 유한요소해석의 연계에 활용이 가능한 제4-5번 요추의 표준 유한요소모델을 개발하였다.
요추 유한요소모델 개발에 적용되는 최신의 모델링 방법을 종합적으로 반영하였으며, Calibration Process를 통해 영역과 방향에 따라 다르게 물성을 적용하여 추간판의 비선형 재료 특성을 구현하였다. 생체 실험과 동일한 하중 조건에서 추간판 내부압력과 운동 가동 범위를 기존의 In vitro 실험 및 타 유한요소 연구 결과와 비교하여 모델을 검증하였으며, 검증 결과는 생체 실험의 측정 범위 내에서 기존 유한요소연구 결과와 전반적으로 일치했다.
유한요소해석의 하중 조건에서 근육 힘과 상체의 중량을 모사하기 위해 실험적 방법에 근거하여 압축 하중으로 작용하는 종동력을 부과하였으며, 실제 척추에 작용하는 주변 근육의 효과가 단순화되어 적용되었다. 또한 본 연구에서는 제4-5번의 단분절 요추에 대해 상세 유한요소모델을 개발하고 순수 압축 하중, 순수 모멘트, 복합 하중의 다양한 하중조건에서 모델을 검증하였으나, 추후 생체활동에 대한 전체 요추의 거동을 분석하기 위해 다분절 요추 유한요소모델의 개발과 후관절(Facet Joint)의 접촉력에 대한 분석이 필요할 것으로 생각된다.
본 연구에서 개발된 제4-5번 요추의 표준 유한요소모델은 전신 인체 유한요소모델의 개발에 적용되어 동작분석 기반의 유한요소해석에 활용할 예정이다. 향후 요추 모델이 포함된 전신 인체 모델은 피험자와 동작에 맞게 변환되며, 동작 분석으로부터 얻어진 운동학적 데이터와 지면 반발력을 하중 조건으로 고려하여 작업 동작 중 추간판의 응력 및 변형을 분석하고 요추 부상의 위험을 예측하는데 활용이 가능할 것으로 기대한다.
Acknowledgments
이 논문은 2020년도 정부(교육부)의 재원으로 한국연구재단의 지원을 받아 수행된 기초연구사업임(No. NRF-2020R1F1A1064012).
REFERENCES
-
Hartvigsen, J., Hancock, M. J., Kongsted, A., Louw, Q., Ferreira, M. L., et al., “What Low Back Pain Is and Why We Need to Pay Attention,” The Lancet, Vol. 391, No. 10137, pp. 2356-2367, 2018.
[https://doi.org/10.1016/S0140-6736(18)30480-X]
-
Hoy, D., Brooks, P., Blyth, F., and Buchbinder, R., “The Epidemiology of Low Back Pain,” Best Practice & Research Clinical Rheumatology, Vol. 24, No. 6, pp. 769-781, 2010.
[https://doi.org/10.1016/j.berh.2010.10.002]
-
Katz, J. N., “Lumbar Disc Disorders and Low-Back Pain: Socioeconomic Factors and Consequences,” The Journal of Bone and Joint Surgery, Vol. 88, pp. 21-24, 2006.
[https://doi.org/10.2106/00004623-200604002-00005]
-
Van Nieuwenhuyse, A., Fatkhutdinova, L., Verbeke, G., Pirenne, D., Johannik, K., et al., “Risk Factors for First-Ever Low Back Pain among Workers in Their First Employment,” Occupational Medicine, Vol. 54, No. 8, pp. 513-519, 2004.
[https://doi.org/10.1093/occmed/kqh091]
- Biomedical, H. D. O. and Science, B., “Work Practices Guide for Manual Lifting,” US Department of Health and Human Services, Public Health Service, Centers, 1981.
-
Waters, T. R., Putz-Anderson, V., Garg, A., and Fine, L. J., “Revised NIOSH Equation for the Design and Evaluation of Manual Lifting Tasks,” Ergonomics, Vol. 36, No. 7, pp. 749-776, 1993.
[https://doi.org/10.1080/00140139308967940]
-
Kim, H. K. and Zhang, Y., “Estimation of Lumbar Spinal Loading and Trunk Muscle Forces during Asymmetric Lifting Tasks: Application of Whole-Body Musculoskeletal Modelling in OpenSim,” Ergonomics, Vol. 60, No. 4, pp. 563-576, 2017.
[https://doi.org/10.1080/00140139.2016.1191679]
-
Brinckmann, P. and Grootenboer, H., “Change of Disc Height, Radial Disc Bulge, and Intradiscal Pressure from Discectomy. An in vitro Investigation on Human Lumbar Discs,” Spine, Vol. 16, No. 6, pp. 641-646, 1991.
[https://doi.org/10.1097/00007632-199106000-00008]
-
Heuer, F., Schmidt, H., Klezl, Z., Claes, L., and Wilke, H. J., “Stepwise Reduction of Functional Spinal Structures Increase Range of Motion and Change Lordosis Angle,” Journal of Biomechanics, Vol. 40, No. 2, pp. 271-280, 2007.
[https://doi.org/10.1016/j.jbiomech.2006.01.007]
-
Panjabi, M. M., Oxland, T., Yamamoto, I., and Crisco, J. J., “Mechanical Behavior of the Human Lumbar and Lumbosacral Spine as Shown by Three-Dimensional Load-Displacement Curves,” The Journal of Bone and Joint Surgery. Vol. 76, No. 3, pp. 413-424, 1994.
[https://doi.org/10.2106/00004623-199403000-00012]
-
Middleton, K. J., Carstairs, G. L., and Ham, D. J., “Lift Performance and Lumbar Loading in Standing and Seated Lifts,” Ergonomics, Vol. 59, No. 9, pp. 1242-1250, 2016.
[https://doi.org/10.1080/00140139.2015.1120888]
-
Ghezelbash, F., Shirazi-Adl, A., Arjmand, N., El-Ouaaid, Z., and Plamondon, A., “Subject-Specific Biomechanics of Trunk: Musculoskeletal Scaling, Internal Loads and Intradiscal Pressure Estimation,” Biomechanics and Modeling in Mechanobiology, Vol. 15, No. 6, pp. 1699-1712, 2016.
[https://doi.org/10.1007/s10237-016-0792-3]
-
Rajaee, M. A., Arjmand, N., Shirazi-Adl, A., Plamondon, A., and Schmidt, H., “Comparative Evaluation of Six Quantitative Lifting Tools to Estimate Spine Loads during Static Activities,” Applied Ergonomics, Vol. 48, pp. 22-32, 2015.
[https://doi.org/10.1016/j.apergo.2014.11.002]
-
Patwardhan, A. G., Havey, R. M., Carandang, G., Simonds, J., Voronov, L. I., et al., “Effect of Compressive Follower Preload on the Flexion-Extension Response of the Human Lumbar Spine,” Journal of Orthopaedic Research, Vol. 21, No. 3, pp. 540-546, 2003.
[https://doi.org/10.1016/S0736-0266(02)00202-4]
-
Rohlmann, A., Bauer, L., Zander, T., Bergmann, G., and Wilke, H. J., “Determination of Trunk Muscle Forces for Flexion and Extension by Using a Validated Finite Element Model of the Lumbar Spine and Measured in vivo Data,” Journal of Biomechanics, Vol. 39, No. 6, pp. 981-989, 2006.
[https://doi.org/10.1016/j.jbiomech.2005.02.019]
-
Park, S., Lee, S., Yoon, J., and Chae, S. W., “Finite Element Analysis of Knee and Ankle Joint during Gait based on Motion Analysis,” Medical Engineering & Physics, Vol. 63, pp. 33-41, 2019.
[https://doi.org/10.1016/j.medengphy.2018.11.003]
-
Lee, S. and Chae, S. W., “Changes in Contact Pressure at the Lower Extremity Joint with an Unstable Shoe,” International Journal of Precision Engineering and Manufacturing, Vol. 20, No. 9, pp. 1611-1619, 2019.
[https://doi.org/10.1007/s12541-019-00162-5]
-
Xu, M., Yang, J., Lieberman, I. H., and Haddas, R., “Lumbar Spine Finite Element Model for Healthy Subjects: Development and Validation,” Computer Methods in Biomechanics and Biomedical Engineering, Vol. 20, No. 1, pp. 1-15, 2017.
[https://doi.org/10.1080/10255842.2016.1193596]
-
Mills, M. J. and Sarigul-Klijn, N., “Validation of an in vivo Medical Image-Based Young Human Lumbar Spine Finite Element Model,” Journal of Biomechanical Engineering, Vol. 141, No. 3, Paper No. 031003, 2019.
[https://doi.org/10.1115/1.4042183]
-
Schmidt, H., Heuer, F., Simon, U., Kettler, A., Rohlmann, A., et al., “Application of a New Calibration Method for a Three-Dimensional Finite Element Model of a Human Lumbar Annulus Fibrosus,” Clinical Biomechanics, Vol. 21, No. 4, pp. 337-344, 2006.
[https://doi.org/10.1016/j.clinbiomech.2005.12.001]
-
Spitzer, V., Ackerman, M. J., Scherzinger, A. L., and Whitlock, D., “The Visible Human Male: A Technical Report,” Journal of the American Medical Informatics Association, Vol. 3, No. 2, pp. 118-130, 1996.
[https://doi.org/10.1136/jamia.1996.96236280]
-
Masharawi, Y., “Lumbar Shape Characterization of the Neural Arch and Vertebral Body in Spondylolysis: A Comparative Skeletal Study,” Clinical Anatomy, Vol. 25, No. 2, pp. 224-230, 2012.
[https://doi.org/10.1002/ca.21203]
-
Pooni, J., Hukins, D., Harris, P., Hilton, R., and Davies, K., “Comparison of the Structure of Human Intervertebral Discs in the Cervical, Thoracic and Lumbar Regions of the Spine,” Surgical and Radiologic Anatomy, Vol. 8, No. 3, pp. 175-182, 1986.
[https://doi.org/10.1007/BF02427846]
-
Hong, C. H., Park, J. S., Jung, K. J., and Kim, W. J., “Measurement of the Normal Lumbar Intervertebral Disc Space Using Magnetic Resonance Imaging,” Asian Spine Journal, Vol. 4, No. 1, pp. 1-6, 2010.
[https://doi.org/10.4184/asj.2010.4.1.1]
-
Sato, F., Odani, M., Endo, Y., Tada, M., Miyazaki, Y., et al., “Analysis of the Alignment of Whole Spine in Automotive Seated and Supine Postures Using an Upright Open MRI System,” International Journal of Automotive Engineering, Vol. 7, No. 1, pp. 29-35, 2016.
[https://doi.org/10.20485/jsaeijae.7.1_29]
-
Naserkhaki, S., Jaremko, J. L., Adeeb, S., and El-Rich, M., “On the Load-Sharing along the Ligamentous Lumbosacral Spine in Flexed and Extended Postures: Finite Element Study,” Journal of Biomechanics, Vol. 49, No. 6, pp. 974-982, 2016.
[https://doi.org/10.1016/j.jbiomech.2015.09.050]
-
Holzapfel, G. A., Schulze-Bauer, C. A., Feigl, G., and Regitnig, P., “Single Lamellar Mechanics of the Human Lumbar Anulus Fibrosus,” Biomechanics and Modeling in Mechanobiology, Vol. 3, No. 3, pp. 125-140, 2005.
[https://doi.org/10.1007/s10237-004-0053-8]
-
Shirazi-Adl, A., Ahmed, A. M., and Shrivastava, S. C., “Mechanical Response of a Lumbar Motion Segment in Axial Torque Alone and Combined with Compression,” Spine, Vol. 11, No. 9, pp. 914-927, 1986.
[https://doi.org/10.1097/00007632-198611000-00012]
-
Schmidt, H., Heuer, F., Drumm, J., Klezl, Z., Claes, L., et al., “Application of a Calibration Method Provides More Realistic Results for a Finite Element Model of a Lumbar Spinal Segment,” Clinical Biomechanics, Vol. 22, No. 4, pp. 377-384, 2007.
[https://doi.org/10.1016/j.clinbiomech.2006.11.008]
-
Lee, C. H., Kim, Y. E., Lee, H. J., Kim, D. G., and Kim, C. H., “Biomechanical Effects of Hybrid Stabilization on the Risk of Proximal Adjacent-Segment Degeneration Following Lumbar Spinal Fusion Using an Interspinous Device or a Pedicle Screw-Based Dynamic Fixator,” Journal of Neurosurgery: Spine, Vol. 27, No. 6, pp. 643-649, 2017.
[https://doi.org/10.3171/2017.3.SPINE161169]
-
Naserkhaki, S., Arjmand, N., Shirazi-Adl, A., Farahmand, F., and El-Rich, M., “Effects of Eight Different Ligament Property Datasets on Biomechanics of a Lumbar L4-L5 Finite Element Model,” Journal of Biomechanics, Vol. 70, pp. 33-42, 2018.
[https://doi.org/10.1016/j.jbiomech.2017.05.003]
-
Schmidt, H., Kettler, A., Heuer, F., Simon, U., Claes, L., et al., “Intradiscal Pressure, Shear Strain, and Fiber Strain in the Intervertebral Disc under Combined Loading,” Spine, Vol. 32, No. 7, pp. 748-755, 2007.
[https://doi.org/10.1097/01.brs.0000259059.90430.c2]
-
Dreischarf, M., Rohlmann, A., Bergmann, G., and Zander, T., “Optimised in vitro Applicable Loads for the Simulation of Lateral Bending in the Lumbar Spine,” Medical Engineering & Physics, Vol. 34, No. 6, pp. 777-780, 2012.
[https://doi.org/10.1016/j.medengphy.2012.04.002]
-
Azari, F., Arjmand, N., Shirazi-Adl, A., and Rahimi-Moghaddam, T., “A Combined Passive and Active Musculoskeletal Model Study to Estimate L4-L5 Load Sharing,” Journal of Biomechanics, Vol. 70, pp. 157-165, 2018.
[https://doi.org/10.1016/j.jbiomech.2017.04.026]
-
Zander, T., Rohlmann, A., and Bergmann, G., “Influence of Different Artificial Disc Kinematics on Spine Biomechanics,” Clinical Biomechanics, Vol. 24, No. 2, pp. 135-142, 2009.
[https://doi.org/10.1016/j.clinbiomech.2008.11.008]
-
Rohlmann, A., Zander, T., Rao, M., and Bergmann, G., “Realistic Loading Conditions for Upper Body Bending,” Journal of Biomechanics, Vol. 42, No. 7, pp. 884-890, 2009.
[https://doi.org/10.1016/j.jbiomech.2009.01.017]
-
Dreischarf, M., Rohlmann, A., Bergmann, G., and Zander, T., “Optimised Loads for the Simulation of Axial Rotation in the Lumbar Spine,” Journal of Biomechanics, Vol. 44, No. 12, pp. 2323-2327, 2011.
[https://doi.org/10.1016/j.jbiomech.2011.05.040]
-
Dreischarf, M., Zander, T., Shirazi-Adl, A., Puttlitz, C., Adam, C., et al., “Comparison of Eight Published Static Finite Element Models of the Intact Lumbar Spine: Predictive Power of Models Improves When Combined Together,” Journal of Biomechanics, Vol. 47, No. 8, pp. 1757-1766, 2014.
[https://doi.org/10.1016/j.jbiomech.2014.04.002]
-
Pearcy, M. J., “Stereo Radiography of Lumbar Spine Motion,” Acta Orthopaedica Scandinavica, Vol. 56, pp. 1-45, 1985.
[https://doi.org/10.3109/17453678509154154]
M.S. candidate in the Department of Mechanical Engineering, Korea University. Her research interest is finite element analysis and motion analysis.
E-mail: minhye_jo@korea.ac.kr
Professor in the School of Mechanical Engineering, Korea University. His research interests include computer aided engineering and human centered design.
E-mail: swchae@korea.ac.kr