
금속 적층 제조된 격자 구조물의 1/4 압축시험 변형 거동 분석
Copyright © The Korean Society for Precision Engineering
This is an Open-Access article distributed under the terms of the Creative Commons Attribution Non-Commercial License (http://creativecommons.org/licenses/by-nc/3.0) which permits unrestricted non-commercial use, distribution, and reproduction in any medium, provided the original work is properly cited.
Abstract
With the development of Additive Manufacturing process, lattice structures have recently been fabricated with fine quality. Lattice structures have unique performances which encompass various elastic responses. In this study, shear characteristics of the lattice structures (BCC and OTC) fabricated by SLM process, under optimized manufacturing conditions, were analyzed by 1/4 compression tests. As a result, several fracture modes and elastic configurations were found by comparing the compression test results of various lattice structures. In addition, the lattice structures possessed certain shear elasticity and normal elasticity among different types of lattices at elastic region when shearing. As the 1/4 compression test was simulating the lattice structure on concentrate load or shearing load, the test represented shock introspection characteristics of the lattice inner structure.
Keywords:
Lattice structure, Additive manufacturing, Elastic configuration키워드:
격자 구조, 적층 제조, 탄성 구성1. 서론
항공, 자동차, 국방 등 다양한 산업분야에서 제품의 경량화를 통한 에너지 효율 증대가 중요한 연구 주제 중 하나이다. 제품의 경량화 방법으로는 위상최적화(Topology Optimization)와 같은 형상 설계기법을 통해 구조 최적화가 일반적이며, 경량 소재의 적용 또는 구조물 내부에 격자 구조(lattice Structure)를 적용한 경량화 방법도 사용한다.1 그 중에서 내부 격자 구조를 이용한 경량화 방법은 샌드위치 패널(Sandwich Panel) 형태로 평판 사이에 격자 구조물을 넣는 방식으로 무게 대비 높은 강도 및 강성, 내충격성 등의 많은 특성을 가지고 있다.2,3
격자 구조는 조인트(Joint)와 스트럿(Strut)으로 단위 셀(Unit Cell)을 구성하고, 전체적인 형상은 육면체로 설계한다. 일반적으로 격자 구조는 스트럿 직경이 균일한 형태로 설계하고, 원하는 강성을 갖도록 설계한다. 이러한 격자 구조는 형상, 셀 사이즈, 스트럿 직경 등에 따라 전체적인 기계적 특성을 다르게 가지게 된다.2,3 최근에 금속 적층 제조(Additive Manufacturing) 공정의 발전으로 미세한 격자 구조 제작이 가능하게 되었다.3-5
격자 구조를 이용한 경량화 연구로는 다음과 같다. Cheng 등은 위상최적화를 통해 상대밀도 분포에 따른 격자 구조 최적화를 진행하여 에너지 전달효율 향상 연구를 진행하였다.6 Han 등은 샌드위치 코어로 격자 구조에 보충재를 사용하여 성능 향상에 대한 연구를 진행하였다.7 또한, Daynes 등은 구조해석을 통해 주응력(Principal Stress) 분포를 이용하여 격자를 배치하는 최적화 연구를 진행하였다.8 Kang 등은 위상최적화 상대밀도(Relative Density) 분포를 통해 다중 격자 구조를 최적화하였고, SLM (Selective Laser Melting) 공정을 통해 제작하여 굽힘 실험으로 그 성능을 평가하였다.9 그 외에도 다양한 격자 구조를 이용한 연구가 진행되었다.10-12
내부 격자 구조의 성능분석에서는 인장, 압축 또는 전단에 대한 단일 특성을 평가지표로 사용하여 설계하였다.13 그러나 실제 격자 내부구조는 복합적인 하중 조건이 주어지기에 그것에 맞는 설계가 중요하다. 따라서 본 연구에서는 기존 연구와 달리 압축과 전단 하중이 동시에 주어지는 조건에서 격자 구조를 해석하고, 실험하는 방법을 제안하였다. 또한 금속 적층 공정을 통하여 제작된 내부 격자를 1/4 압축시험법(Quarter Compression Test, QCT)을 제시하여 압축과 전단 특성을 동시에 평가하였다. 본 연구에 사용된 격자 구조는 BCC (Body Centered Cubic) 구조와 OTC (Octet-Truss Cubic)를 사용하였다.
2. 시편 설계 및 제작
2.1 격자 구조 설계
격자 형상은 Fig. 1에서 나타낸 단위 셀 형태의 BCC와 OTC 구조를 사용했다.11,14 단위 셀 크기는 미세 격자 구조의 제작 가능한 한 변의 길이가 2 mm인 육면체 형태로 설계하였다. 기하학적 분류로서 Maxwell의 안정성 기준(Maxwell’s Stability Criterion)에 의해 굽힘변형 지배적 구조와 인장변형 지배적 구조로 분류된다.14 굽힘변형 지배적 구조는 탄성변형의 폭이 크지만 강성이 낮고, 인장변형 지배적 구조는 강성, 강도가 높지만 변형 폭이 제한적인 특징이 있다. Figs. 1(a)의 BCC 구조는 굽힘변형 지배적 구조이고, 1(b)의 OTC는 인장변형 지배적 구조로 분류된다. 일반적인 전면 압축 실험과 1/4 압축 실험을 위해서 Figs. 2(a)와 2(b)와 같이 격자 구조 시편을 설계하였다. 5층의 단위 격자가 높이 방향으로 배치되고 위 아래에 0.5 mm 평판을 배치하였다.
격자 구조의 스트럿 직경(D)은 Kang 등의 기존 연구를 참고하여 0.3, 0.4, 0.5 mm로 설계했다.2,9,15,16 제작성을 고려해 0.3 mm를 스트럿의 최소 직경으로 하였다. 스트럿 최대 직경은 적층 제조로 제작된 시편에서 잔류한 금속 분말이 토출 될 수 있는 공간이 확보되는 0.5 mm로 설계하였다. 잔류 분말이 토출 될 수 있는 최소 갭(DP)는 형상이 복잡한 OCT 구조를 기반으로 설계되며 아래 식(1)과 식(2)로 표현하였다.
(1) |
(2) |
여기서 DPOTC는 최소 갭 직경이고, L은 셀 크기, Dpow는 파우더 입도를 나타낸다.
2.2 시편 제작
시편 제작에 사용된 금속 적층공정 장비는 ProX-300(3D Systems, USA)이다. 금속 파우더는 SUS-630를 사용하였으며, 평균 입도는12.93 μm이다. SLM 공정 조건으로 레이저 출력은 170W, 레이저 스캔 속도는 1.6 m/s이다. 보호가스로는 아르곤(Ar)을 사용하였으며, 적층 두께는 0.2 mm로 하였다. 후처리 공정으로 490oC에서 2시간 동안 어닐링(Annealing)을 실시하여 적층 제조 공정 중에 발생한 잔류응력을 제거하였다.
적층 제조를 통해 제작된 시편은 Fig. 3에 나타내었다. 스트럿 직경이 0.3 mm인 OTC 구조의 경우 스트럿 간의 연결부에서 제작이 되지 않아 압축 실험 시편에서 제외하였다.
3. 압축 실험
3.1 실험 구성
본 연구에서 제안된 QCT 실험은 재료시험기(RB-310, R&B Co., Korea)를 사용한다. Fig. 4(a)에서 나타낸 것처럼 QCT 실험은 시편의 1/4 부분만을 압축하는데 압축되는 부분과 나머지 부분 사이에 전단변형 영역이 발생하게 되어 QCT 실험 방법은 압축과 전단변형으로 모두 관찰할 수 있는 방법이다. 또한 일반적인 전면 압축 실험도 실시하여 격자 구조의 재료 거동을 비교하였다. QCT 실험의 경우 특별한 고정 지그가 필요한데 Fig. 4(b)에 나타낸 것처럼 시편이 압축되는 동안 변형 거동을 방해하지 않도록 폭 방향의 변형이 자유롭게 일어나도록 설계하였다.
1/4 압축 실험은 압축 부분에 대한 이해, 또한 압축 부분과 비압축 부분 사이의 전단변형이 발생되는 부분에 대한 이해를 하기 위한 것이다. 실험은 격자 구조의 파단변형이 발생할 때까지 진행했으며 시편 단면이 1/4 형상(5 × 5 mm)만을 압축하게 된다. 그리고 ASTM E9-89a 실험규격에 따라 압축 속도가 0.5 mm/min로 진행하였다.
3.2 구조해석
1/4 실험에서 격자 구조의 탄성 거동을 분석하기 위해 유한요소해석을 진행하였다. 해석에는 ABAQUS (Ver. 14.4)를 사용하였고, 요소(Element)는 사면체 요소를 사용하였으며 평균 요소 크기는 0.2 mm로 두었다. 또한, 해석은 Fig. 3에 나타낸 5가지 시편 모델링에 1/4 압축 조건으로 변형량이 1 mm가 되는 시점까지 선형해석을 진행하였다. 해석에 사용된 소재는 SUS630이며, 탄성계수는 195 GPa, 포아송비 0.27이다.
Figs. 5(a)부터 5(e)에 나타낸 해석 결과는 1/4 압축 조건에서 1 mm 변형일 때 응력분포이다. 스트럿 직경(D)이 클수록 동일한 변형량을 발생시키기 위한 하중이 많이 걸리며, 이에 따라 응력도 증가함을 알 수 있다.
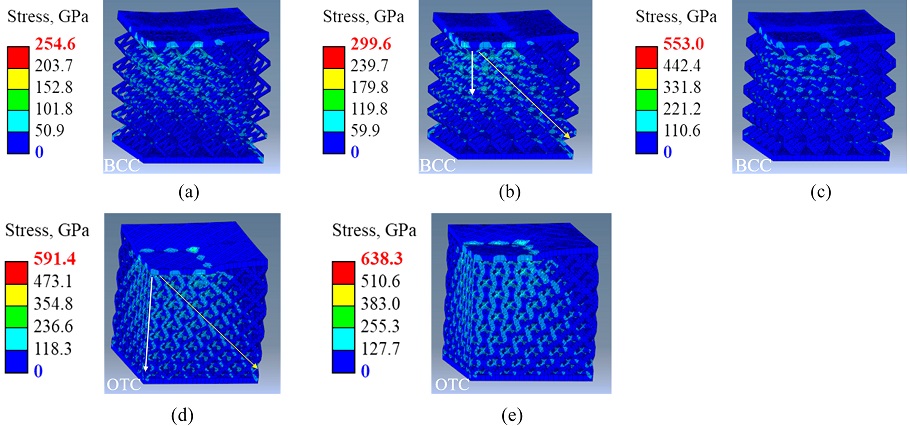
Finite element analysis of quarter compression test on the model: BCC with (a) D = 0.3 mm, (b) D = 0.4 mm, (c) D = 0.5 mm and OTC with (d) D = 0.4 mm, and (e) D = 0.5 mm
동일한 스트럿 직경(D = 0.4 mm)을 가진 BCC와 OTC 구조를 비교할 때, 1 mm 변형 발생에 걸리는 하중은 OTC 격자 구조가 BCC 구조에 비하여 1.97배 증가하였다. 따라서 OTC 구조가 압축변형에 대한 저항이 큰 것으로 나타났다.
압축면과 비압축면 사이에 전단변형 구간이 나타나며, OTC 구조가 BCC 구조에 비하여 Figs. 5(b)와 5(d)에 나타낸 것처럼 시편의 전체적으로 힘의 전달 범위가 넓고, 응력이 분포적으로 걸리는 것을 알 수 있다. 전단변형이 발생하는 구간을 보면 BCC 구조와 OTC 구조 모두에서 힘을 받는 1/4 영역 부분을 중심으로 45° 방향으로 힘전달이 발생하고 있다. 따라서 1/4 압축 실험이 압축변형과 전단변형이 동시에 관찰되고, 설계된 격자 구조에서 두 방향의 강성을 알 수 있는 방법임을 해석적으로 증명하였다.
3.3 실험 결과
본 실험은 SLM 공정으로 제작된 격자 구조의 기계적 변형 거동 및 파단 특성을 관찰하기 위하여 실시하였다. 실험은 전면 압축 실험과 QCT 실험으로 총 2가지 실험으로 구성되었다. 실험 결과로는 전면 압축 실험에서 하중(Fp)과 변위(dp)를 측정하여 하중-변위 곡선을 Figs. 6(a)와 같이 표시하였고, 1/4 압축 실험(QCT)에서는 격자 구조가 부분적인 압축으로 압축과 전단의 탄성이 혼재된 하중(Fq)과 변위(dq)를 측정하여 하중-변위 그래프를 6(b)와 같이 표시하였다.
격자 구조가 압축될 때의 성능을 평가하는 성능지표로서 압축탄성(Ep)을 식(3)과 같이 정의하였다. QCT 실험은 전면 압축 실험과 사용된 시편이 동일하고, 격자 구조의 시편 특성상 압축탄성은 병렬 연결되기 때문에 QCT 실험에서의 압축탄성(Ep')을 식(4)와 같이 정의하였다. 또 압축과 전단의 작용점이 다르다고 판단하고, 이로 인해 발생하는 반력 또한 독립적으로 계산하여 QCT 실험에서 전단변형 특성 지표인 전단탄성(Es')을 식(5)와 같이 표시하였다.
(3) |
(4) |
(5) |
위 2가지 성능지표(Ep',Es)를 실험 결과를 통하여 아래 Fig. 7과 같이 정리하였고, 실험 결과와 더불어 3.2절에서 계산한 해석 결과도 같이 정리하였다. 그리고 실험에서 2가지 형상의 파단 과정을 Figs. 8(a)와 8(b)를 통해 표시하였는데, 변위가 0.5 mm마다 최대 2 mm까지의 파괴되는 시편을 표시하였다. 시편은 격자별로 전단과 압축변형 모두 발생하였고, 파단 과정에서 좌굴된 격자가 강도 보강이 되는 형태로 파단이 발생하기도 하였다.15
실험 결과에서 격자 구조의 형상과 스트럿 직경을 떠나 부분 압축 상황에서 모든 시편의 전단탄성은 평균 76.9%를 차지하고, 시편간 최대 편차량은 4.1%이다. 해석 결과에서도 모든 시편의 전단탄성은 평균 56.5%이며, 최대 편차량은 2.7%이다. 실험과 해석 모두 격자 구조는 형상과 스트럿 직경(상대밀도)를 별개로 전단탄성이 차지하는 비율이 일정하며, QCT 압축의 경우 전단탄성이 지배적으로 판단된다.
Fig. 9(a)에는 변형된 시편의 형태를 나타내었는데, 절단된 단면을 SEM을 활용하여 촬영하였다. Fig. 9(b)는 전반전인 전단면을 찍은 장면인데, 변위가 다른 α, β, γ 영역을 확대하여 Fig. 9의 (α), (β), (γ)과 같이 표시하였다. 전단면을 관찰한 결과, 우선 인장에 의한 파단과 전단에 의한 파단을 파괴면의 형상을 보고 추측할 수 있는데, 인장에 의해 파단된 단면은 파괴면의 높낮이가 일정하지 않고, 재료의 계면이 비교적 명확하게 관찰된다. 그와 반대로 전단에 의해 파단된 단면은 파괴면의 높낮이가 일정하고, 파괴된 방향을 따라 재료가 이동한 흔적이 남으며 재료의 계면을 구분하기 어려운 형상으로 관찰된다. Fig. 9의 (α), (β), (γ)는 순차적으로 변위가 줄어드는 순서로 배치하였는데, 변위가 감소할수록 전단에 의한 파괴가 감소하고, 인장에 의한 파괴가 증가하는 것을 관찰할 수 있었다.18,19 이는 3.2절의 해석 결과와 같이 Figs. 9(α)처럼 변위가 큰 영역은 파괴될 때 압축에 의한 응력이 전단면에 전달이 거의 안되는 반면, 9(γ)와 같이 변위가 작은 영역은 압축에 의한 응력이 충분히 전달되어 변형 방향에 상응하는 방향으로 압축응력이 걸린다.17,20 따라서 전단에 의한 파괴는 상부에서 하부로 변위가 감소할수록 지배적인 위치에서 점차 감소하는 것으로 판단된다.13,21
4. 결론
본 연구에서는 SLM 공정을 통해 BCC, OTC 구조 각각 다른 스트럿 직경의 시편을 제작하였고, 전면 압축 실험, QCT 실험, 구조해석을 진행하였다. 또 실험을 통해 시편의 파단 과정을 관찰하고, 파단면에 SEM 촬영을 통해 다음과 같은 결론을 도달하였다.22
첫째, 격자 구조는 QCT 실험에서 전단과 압축에 의한 변형이 모두 발생하였는데, 이는 부분적인 하중 조건에서 격자 구조의 성능은 압축탄성과 전단탄성 모두 기여한다. 둘째, 변형에 대한 기여도에서 시편 종류를 막론하고 전단탄성이 실험과 해석 모두 지배적으로 나타났다. 따라서 부분적인 압축 하중을 받는 격자 구조를 설계할 때 전단에 대한 영향을 잘 고려할 필요가 있다. 셋째, 전단탄성이 격자 구조 성능에 기여하는 비율이 실험과 해석에서 모두 유사하고 실험에서는 4.1, 해석에서는 2.7%의 편차범위 이내의 값을 가진다. 넷째, 파단면의 SEM 촬영을 통하여 파단 과정 중 변위에 따라 인장에 의한 파괴가 증가하지만 전단에 의한 파단면이 지배적이고, 실험과 해석에서 모두 전단탄성이 격자 구조 성능에 지배적인 기여를 하기 때문에 파단 또한 전단에 의한 파단이 지배적으로 판단된다. 본 연구에서 제안한 QCT 실험은 격자 구조의 부분적 압축 하중 하에서의 변형 거동과 파단을 관찰할 수 있으며 압축과 전단 거동에 대한 특성을 분석할 수 있다.
NOMENCLATURE
M : | Maxwell’s Stability Criterion |
b : | Number of Struts |
j : | Number of Joints |
ST : | Stiffness of Total Structure |
dc : | Load Displacement of Compression Test |
Nclash : | Times of Clash Enhancement |
Nlaver : | Number of Lattice Structure Layers |
Acknowledgments
본 연구는 한국연구재단 지원과제(Nos. 2019R1A5A8083201 & 2020R1F1A106937412) 지원으로 수행하였습니다.
REFERENCES
-
Gorguluarslan, R. M., Park, S. I., Rosen, D. W., and Choi, S. K., “A Multilevel Upscaling Method for Material Characterization of Additively Manufactured Part under Uncertainties,” Journal of Mechanical Design, Vol. 137, No. 11, Paper No. 111408, 2015.
[https://doi.org/10.1115/1.4031012]
-
Xu, Y., Zhang, D., Hu, S., Chen, R., Gu, Y., et al., “Mechanical Properties Tailoring of Topology Optimized and Selective Laser Melting Fabricated Ti6Al4V Lattice Structure,” Journal of the Mechanical Behavior of Biomedical Materials, Vol. 99, pp. 225-239, 2019.
[https://doi.org/10.1016/j.jmbbm.2019.06.021]
-
Leary, M., Mazur, M., Elambasseril, J., McMillan, M., Chirent, T., et al., “Selective Laser Melting (SLM) of AlSi12Mg Lattice Structures,” Materials & Design, Vol. 98, pp. 344-357, 2016.
[https://doi.org/10.1016/j.matdes.2016.02.127]
-
Kim, D. I., Lee, H. J., Ahn, D. G., Kim, J. S., and Kang, E. G., “Preliminary Study on Improvement of Surface Characteristics of Stellite21 Deposited Layer by Powder Feeding Type of Direct Energy Deposition Process Using Plasma Electron Beam,” Journal of the Korean Society for Precision Engineering, Vol. 33, No. 11, pp. 951-959, 2016.
[https://doi.org/10.7736/KSPE.2016.33.11.951]
-
Meyer, G., Brenne, F., Niendorf, T., and Mittelstedt, C., “Influence of the Miniaturisation Effect on the Effective Stiffness of Lattice Structures in Additive Manufacturing,” Metals, Vol. 10, No. 11, Paper No. 1442, 2020.
[https://doi.org/10.3390/met10111442]
-
Cheng, L., Zhang, P., Biyikli, E., Bai, J., Robbins, J., et al., “Efficient Design Optimization of Variable-Density Cellular Structures for Additive Manufacturing: Theory and Experimental Validation,” Rapid Prototyping Journal, Vol. 23, pp. 660-677, 2017.
[https://doi.org/10.1108/RPJ-04-2016-0069]
-
Han, B., Zhang, Z. J., Zhang, Q. C., Zhang, Q., Lu, T. J., et al., “Recent Advances in Hybrid Lattice-Cored Sandwiches for Enhanced Multifunctional Performance,” Extreme Mechanics Letters, Vol. 10, pp. 58-69, 2017.
[https://doi.org/10.1016/j.eml.2016.11.009]
-
Daynes, S., Feih, S., Lu, W. F., and Wei, J., “Optimisation of Functionally Graded Lattice Structures Using Isostatic Lines,” Materials & Design, Vol. 127, pp. 215-223, 2017.
[https://doi.org/10.1016/j.matdes.2017.04.082]
-
Kang, D., Park, S., Son, Y., Yeon, S., Kim, S. H., et al., “Multi-Lattice Inner Structures for High-Strength and Light-Weight in Metal Selective Laser Melting Process,” Materials & Design, Vol. 175, Paper No. 107786, 2019.
[https://doi.org/10.1016/j.matdes.2019.107786]
-
Woischwill, C. and Kim, I. Y., “Multimaterial Multijoint Topology Optimization,” International Journal for Numerical Methods in Engineering, Vol. 115, No. 13, pp. 1552-1579, 2018.
[https://doi.org/10.1002/nme.5908]
-
Liu, J., Gaynor, A. T., Chen, S., Kang, Z., Suresh, K., et al., “Current and Future Trends in Topology Optimization for Additive Manufacturing,” Structural and Multidisciplinary Optimization, Vol. 57, No. 6, pp. 2457-2483, 2018.
[https://doi.org/10.1007/s00158-018-1994-3]
-
Liu, C., Du, Z., Zhang, W., Zhu, Y., and Guo, X., “Additive Manufacturing-Oriented Design of Graded Lattice Structures through Explicit Topology Optimization,” Journal of Applied Mechanics, Vol. 84, No. 8, Paper No. 081008, 2017.
[https://doi.org/10.1115/1.4036941]
-
Kim, Y., Kim, Y., and Ryu, S., “Elastic and Fracture Property Analyses of Triangular and Square Lattice Spring Models at a Large Deformation Regime,” Journal of Mechanical Science and Technology, Vol. 32, No. 6, pp. 2693-2706, 2018.
[https://doi.org/10.1007/s12206-018-0526-9]
-
Deshpande, V., Ashby, M., and Fleck, N., “Foam Topology: Bending Versus Stretching Dominated Architectures,” Acta Materialia, Vol. 49, No. 6, pp. 1035-1040, 2001.
[https://doi.org/10.1016/S1359-6454(00)00379-7]
-
Ye, J. Q., Kang, D. S., Yeon, S. M., Son, Y., and Park, S. H., “Analysis on Deformation Behavior and Restructuring of Additive Manufactured Metal Lattice-Structures under Compressive Loading Condition,” Journal of the Korean Society for Precision Engineering, Vol. 37, No. 8, pp. 625-631, 2020.
[https://doi.org/10.7736/JKSPE.020.015]
-
Jin, Q. Y., Yu, J. H., Ha, K. S., Lee, W. J., and Park, S. H., “Multi-Dimensional Lattices Design for Ultrahigh Specific Strength Metallic Structure in Additive Manufacturing,” Materials & Design, Vol. 201, Paper No. 109479, 2021.
[https://doi.org/10.1016/j.matdes.2021.109479]
-
Nurbanasari, M., “Crack of a First Stage Blade in a Steam Turbine,” Case Studies in Engineering Failure Analysis, Vol. 2, No. 2, pp. 54-60, 2014.
[https://doi.org/10.1016/j.csefa.2014.04.002]
-
Jung, M. H., Kong, J. R., and Kim, H. J., “Dimensional Characteristics of 3D Printing by FDM and DLP Output Methods,” Journal of the Korean Society of Manufacturing Process Engineers, Vol. 20, No. 1, pp. 66-73, 2021.
[https://doi.org/10.14775/ksmpe.2021.20.01.066]
-
Lee, J. S., Choi, B. J., Lee, M. G., Kim, J. S., Lee, S. W., et al., “Defect Classification of Cross-Section of Additive Manufacturing Using Image-Labeling,” Journal of the Korean Society of Manufacturing Process Engineers, Vol. 19, No. 7, pp. 7-15, 2020.
[https://doi.org/10.14775/ksmpe.2020.19.07.007]
-
Unigovski, Y. B., Lothongkum, G., Gutman, E., Alush, D., and Cohen, R., “Low-Cycle Fatigue Behavior of 316l-Type Stainless Steel in Chloride Solutions,” Corrosion Science, Vol. 51, No. 12, pp. 3014-3020, 2009.
[https://doi.org/10.1016/j.corsci.2009.08.035]
-
Schlangen, E. and Garboczi, E. J., “Fracture Simulations of Concrete Using Lattice Models: Computational Aspects,” Engineering Fracture Mechanics, Vol. 57, Nos. 2-3, pp. 319-332, 1997.
[https://doi.org/10.1016/S0013-7944(97)00010-6]
-
Yi, H. W., Lee, Y. U., Lee, M. W., Kwon, J. Y., and Kang, M., “Analysis and Evaluation of Separation Efficiency on Mass Flow of Mini Hydro Cyclone Separator Manufactured by 3D Printing,” Journal of the Korean Society of Manufacturing Process Engineers, Vol. 20, No. 7, pp. 89-96, 2021.
[https://doi.org/10.14775/ksmpe.2021.20.07.089]
Ph.D. candidate in the Department of Mechanical Engineering, Pusan National University. His research interest is design of lattice structure with topology optimization.
E-mail: sub6953@kitech.re.kr
Researcher in Korea Institute of Industrial Technology (KITECH). His research interest is additive manufacturing process.
E-mail: simo@kitech.re.kr
Principal researcher in Korea Institute of Industrial Technology (KITECH). His research interest is additive manufacturing process.
E-mail: sonyong@kitech.re.kr
Professor in School of Mechanical Engineering, Pusan National University. His research interest is additive manufacturing process and DfAM. He is a member of directors board in KSME, KSPE, and KSMPE.
E-mail: sanghu@pusan.ac.kr