
전압 범위에 따른 활성화 영향성 평가

Copyright © The Korean Society for Precision Engineering
This is an Open-Access article distributed under the terms of the Creative Commons Attribution Non-Commercial License (http://creativecommons.org/licenses/by-nc/3.0) which permits unrestricted non-commercial use, distribution, and reproduction in any medium, provided the original work is properly cited.
Abstract
Polymer electrolyte membrane fuel cells (PEMFC) require activation to maximize their performance. Thus, an appropriate activation process is essential for the performance of the fuel cell. In this study, the performance of the fuel cell was investigated by changing the voltage range during the activation process. There were three voltage ranges: 0.3-0.9 V, 0.3-0.6 V, and 0.6-0.9 V. When the fuel cell was activated in the low voltage region, the highest performance was output. On the other hand, it showed the lowest performance at high voltage. The results suggest that it is advantageous to activate the fuel cell with a high current. On the other hand, if activation is performed while outputting at a low current, the generation of water and the electrochemical reaction are insufficient, resulting in a load on the fuel cell. Through this experiment, it was confirmed that the control method greatly affects fuel cell performance when activated.
Keywords:
Activation, Nafion, Catalyst layer, Hydration, Electrochemical reaction키워드:
활성화, 나피온, 촉매, 수화, 전기화학반응1. 서론
과거부터 지금까지 주 에너지원으로 화석 연료를 사용했다. 화석 연료를 사용하면서 많은 양의 탄소 배출로 인해 환경오염을 일으키고 이상기후 현상이 발생하고 있다. 따라서 현재 탄소배출을 규제하여 화석 연료 사용량을 줄이고 차 선택으로 신재생에너지를 사용하는 것이 대두되고 있다. 수소는 핵융합발전 및 연료전지 등 많은 산업에 적용되고 있다. 특히 수소를 에너지원으로 사용하는 연료전지는 종류가 다양한데 그 중 고분자 전해질 연료전지(Polymer Electrolyte Membrane Fuel Cell, PEMFC)는 얇은 고분자 막을 전해질로 사용하는 연료전지이다[1-3]. PEMFC는 낮은 온도에서 작동하며 높은 전력 밀도를 갖기 때문에 차량 및 선박과 같은 운송이나 발전기 등 다양한 분야에서 사용할 수 있다[4-7]. PEMFC는 수소와 공기 또는 산소만을 사용하기 때문에 탄소 배출이 없으며 오직 물(H2O)을 배출한다.
PEMFC는 크게 세 부분으로 수소가 들어가는 연료극(Anode), 수소 이온이 이동하는 전해질(Electrolyte), 공기가 들어가는 공기극(Cathode)으로 이루어져 있다. 이때 전극과 전해질을 합친 것이 막전극접합체(Membrain Electrode Assembly, MEA)로 PEMFC에서 가장 핵심적인 부품이다.
PEMFC의 성능을 극대화하기 위해 MEA의 활성화(Activation)과정이 필요하다. 활성화 과정은 PEMFC의 전해질인 나피온(Nafion)을 수화(Hydration)시켜 양성자전도도를 향상시킨다[1,8-11]. 또한, 전기화학반응(Electrochemical)을 통해 백금 촉매의 오염물질을 제거하고 전극 구조 변형을 통해 물질의 반응속도를 개선한다[12,13].
활성화 방법에는 다양하다. 전해질인 나피온의 수화 측면에서 살펴보면 Qi et al.은 전극을 물에 끓이거나 가정용 압력솥에서 쪘다[14]. 또한, 전기화학반응 측면에서 살펴보면 Silva et al. 은 25oC에서 6개의 부하 사이클을 거친 후 연료전지 성능을 평가했다[15]. Yuan et al.은 정전압 0.6 V에서 활성화 작업을 진행한 후 전기화학적 임피던스 분석과 순환 전압 전류법을 통해 MEA의 성능을 확인했다[16]. Zhiani et al.은 세 가지의 활성화 절차에 대한 영향을 비교했는데 하나는 정전압 0.6 V에서 활성화 영향, 두 번째는 0.7 V와 0.5 V 사이의 전압 순환으로 진행한 활성화 영향, 마지막으로 12시간 동안 정전류 0.25 A/cm2에서 활성화 영향을 살펴보았다[17]. 외에도 전극의 열처리 방법도 있었는데 Bezerra et al.은 열처리를 통해 Pt/C 촉매를 활성화하여 산소환원반응(Oxygen Reduction Reaction, ORR)을 향상할 수 있다고 했다[18].
본 연구의 목적은 연료전지의 활성화 작동전압을 크게 세 가지로 분류하여 활성화 작업을 진행하고 각각의 성능을 비교하여 더 높은 성능을 출력하는 활성화 전압 범위를 찾는 것이다. 이때 작동전압은 전 범위(0.3-0.9 V), 저전압 영역(0.3-0.6 V), 고전압 영역(0.6-0.9 V) 세 가지로 분류했다.
2. 실험
2.1 실험 장비
Fig. 1은 실험 장치의 시스템을 보여주고 있다. Phocos 사의 질량유량계(Mass Flow Controllers, MFC)를 이용하여 수소와 공기를 셀(Cell)에 공급하였다. 이때 질량유량계로 들어가는 기체의 압력은 각각 0.15 MPa로 공급되었다. 유량은 수소 100 sccm, 공기 500 sccm으로 설정했다. 가습(Humidification)은 공기극에만 진행했으며 증류수(De-ionized Water, DI Water)로 막 가습기를 이용하여 진행했다. 셀 온도는 25oC에서 실험이 이루어졌다. 셀에서 발생하는 전기화학적 분석을 위해 BioLogic 사의 포텐 시오스텟(SP-150)과 부스터(VMP3B-20)를 이용하여 측정했다.
PEMFC 셀의 분리판(Bipolar Plates)은 사형유로(Serpentine Channels) 구조를 가진 그라파이트(Graphite) 분리판을 이용했으며 유로의 깊이는 0.8 mm, 폭은 1.0 mm이다. 또한, 기체 누설을 방지하는 CNL Energy 사의 가스켓(TF Gaskets), 다공성 구조를 가진 SGL Carbon 사의 기체 확산층(Gas Diffusion Layers, GDL 39 BB), 전극과 전해질로 구성된 CNL Energy 사의 막전극 접합체를 사용했다. 본 연구에서 셀의 활성화 면적은 5 cm2이다.
2.2 실험 방법
우선 엔드 플레이트(End Plate)로 PEMFC 셀의 구성품들을 볼트와 너트를 이용하여 고정했다. 이때 볼트 체결 토크(Torque)는 80 lb·in이다. 셀 조립 후 활성화 과정을 진행하기 전에 셀 조립 상태를 확인하였다. 이는 개방회로전압(Open Circuit Voltage, OCV)을 5분 측정하는 방법으로 이루어졌다. 이후 활성화 과정을 진행했는데 크게 세 가지 방법으로 진행했다. 첫 번째는 전압을 0.3 V부터 0.9 V까지 총 200번 분극 곡선(Polarization Curves)을 그리면서 진행했다. 두 번째는 0.3 V부터 0.6 V까지 200번 분극 곡선을 그렸다. 세 번째는 0.6 V부터 0.9 V까지 마찬가지로 200번 분극 곡선을 그렸다. 활성화 과정 이후 셀의 성능을 확인하기 위해 전압 범위 0.3 V부터 0.9 V까지 연속적으로 제어하면서 전압–전류의 관계를 살펴보았다. 또한, PEMFC에서 발생하는 저항으로 인한 손실(Loss)을 분석하기 위해 0.5 V 전압에서 전기화학적 임피던스 분석(Electrochemical Impedance Spectroscopy, EIS)을 진행했다.
3. 결과 및 고찰
3.1 전압 범위에 따른 활성화된 연료전지 성능
Fig. 2(a)는 각 전압 범위로 제어하면서 활성화 과정을 거친 후 연료전지 셀의 성능을 보여주는 곡선이다. 가장 높은 출력은 활성화 작업 시 0.3-0.6 V 전압 범위에서 진행한 것이며 가장 낮은 성능은 0.6-0.9 V에서 진행한 경우이다. Table 1과 Fig. 2(b)를 살펴보면 0.3-0.6 V에서 활성화 과정을 거친 경우 연료전지가 출력하는 최대 전력 밀도는 648.6 mW/cm2이다. 이는 전 범위 0.3-0.9 V에서 진행한 경우보다 약 8.35% 증가한 것으로 나타났다. 반면 고전압인 0.6-0.9 V에서 활성화 작업을 진행한 셀이 출력하는 최대 전력밀도가 430.4 mW/cm2으로 전 범위로 진행한 경우보다 28.1% 감소한 것을 확인할 수 있다.
Fig. 3(a)는 활성화 과정 동안 연료전지에서 출력되는 전류밀도를 보여주고 있다. 활성화 작업 시간은 같으며 전압 범위가 0.3-0.9 V와 0.3-0.6 V인 경우 활성화 작업을 진행함에 따라 출력되는 전류밀도가 높아지는 것을 확인할 수 있다. 반면 전압 범위가 0.6-0.9 V인 경우 출력되는 전류밀도가 시간이 지남에 따라 오히려 감소하는 모습을 볼 수 있다. Fig. 3(b)와 Table 2는 각 경우에 대해 초기 연료전지가 출력하는 전류밀도에 활성화 마지막 부분에서 연료전지가 출력하는 전류밀도를 비교한 것이다. 0.3-0.9 V에서 활성화 작업을 진행한 경우 최종 전류밀도가 초기 출력되는 전류밀도보다 30.05% 증가한 것을 확인할 수 있다. 또한, 0.3-0.6 V에서 활성화 작업을 진행한 경우 최종 전류 밀도가 초기보다 37.42% 증가한 것을 알 수 있다. 반면 0.6-0.9 V에서 활성화 작업이 이루어진 셀은 최종 전류밀도가 초기값보다 18.56% 감소하여 오히려 연료전지에 부하가 걸린 모습을 확인할 수 있다. 이는 공급되는 수분의 양은 같으나 전기화학반응 측면에서 살펴보았을 때 저전압에서 활성화 작업을 진행하는 경우 더 많은 전하가 이동하고 이로 인해 충분한 물이 생성되어 나피온에 수화를 일으키고 연료전지의 성능을 개선한 것을 확인할 수 있다. 또한, 더 많은 전기화학 반응을 통해 백금 촉매의 오염도가 감소하여 성능이 개선된 것으로 추정된다[12]. 반면 고전압에서 활성화를 진행한 경우 생성되는 물의 양이 부족하여 충분한 수화가 이루어지지 못하고 동시에 백금 촉매의 오염물질을 충분히 제거할 수 없었기 때문에 고전압에서의 활성화 과정이 오히려 연료전지에 부하로 작용한 것을 살펴볼 수 있다.
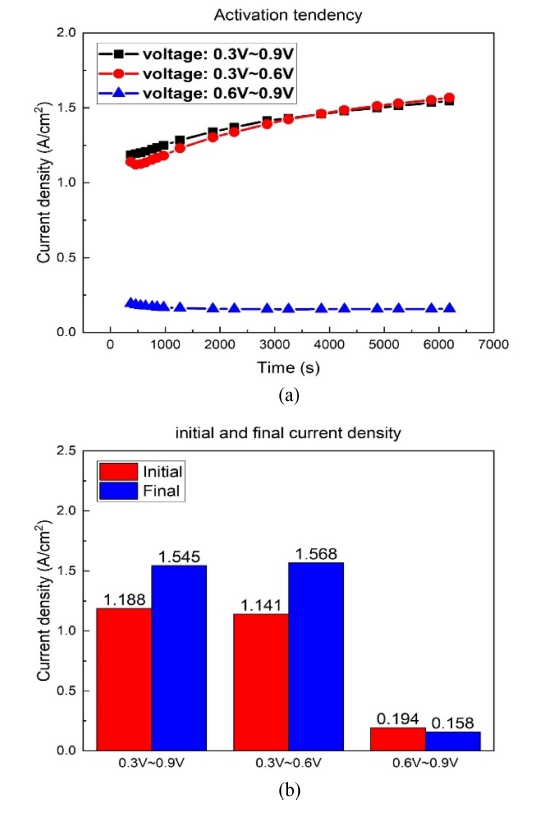
PEMFC performance during the activation process (a) Current density as a function of the activation time (b) Comparison of current density at the initial and final stages of the activation
3.2 전압 범위에 따른 활성화된 연료전지 임피던스
Fig. 4(a)는 연료전지에서 최대 전력 밀도 영역에서 발생하는 임피던스를 살펴보기 위해 0.5 V 전압에서 EIS를 측정한 결과이다. 일반적으로 원점으로부터 반원의 시작점까지의 크기는 Ohmic Resistance이고 반원의 시작점부터 끝나는 지점까지의 크기를 Activation Resistance이다. Activation Resistance는 크게 두 가지로 반원 시작부터 주파수가 1 kHz 부근까지의 반원의 크기는 Anode 전극에서의 Activation Resistance이고 이후 반원의 끝 지점까지의 크기를 Cathode 전극에서의 Activation Resistance이다. 0.6-0.9 V인 경우가 가장 큰 반원을 갖고 동시에 반원의 초기지점이 원점으로부터 가장 멀리 떨어져 있다. 반면 저전압인 0.3-0.6 V인 경우는 가장 작은 반원의 모습과 반원의 초기지점과 원점 사이의 거리가 가장 가까운 것을 볼 수 있다. Figs. 4(b)와 4(c)를 통해 각 경우에 대해 자세한 저항 값을 살펴볼 수 있다.
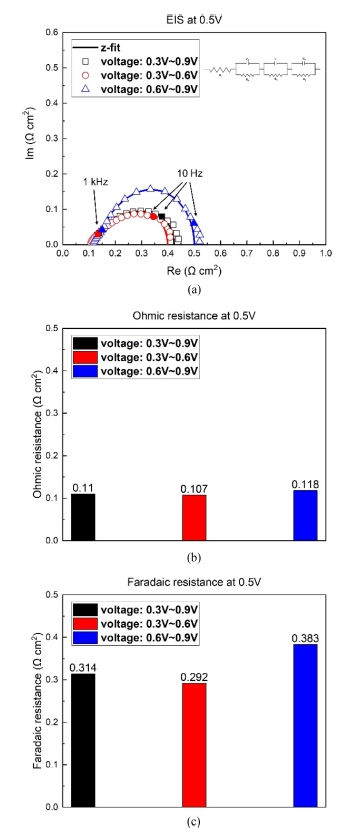
Comparison of EIS curves, ohmic resistance, activation resistance of fuel cells (a) Nyquist plot at 0.5 V (b) Ohmic resistance at 0.5 V (c) Activation resistance at 0.5 V
Fig. 4(b)를 살펴보면 각 전압 범위에서 활성화한 경우에 따라 Ohmic Resistance를 보여주고 있다. 저전압에서 진행한 경우 전 범위로 활성화 작업을 진행한 것보다 2.73% 감소한 것을 알 수 있다. 반면 고전압에서 진행한 경우 전 범위 경우보다 7.27% 증가한 것을 확인할 수 있다. 이를 통해 저전압에서 활성화를 진행한 경우 더 많은 물의 생성으로 인해 Ohmic Resistance가 감소한 것을 알 수 있으며 고전압에서 활성화를 진행한 경우 적은 물로 나피온에 충분한 수화가 발생하지 않아 Ohmic Resistance가 전 범위에 비해 급격하게 증가한 것을 알 수 있다.
Fig. 4(c)는 연료전지에서 발생하는 Activation Resistance를 보여주고 있다. 저전압에서 활성화 작업을 진행한 연료전지는 전 범위에서 진행한 것보다 7.01% Activation Resistance가 감소한 것을 알 수 있다. 반면 고전압에서 활성화 작업을 진행한 셀은 전 범위에서 진행한 것보다 21.97% 증가한 것을 알 수 있다. 이는 저전압에서 활성화 과정을 진행한 것이 더 활발한 전기화학 반응으로 인해 백금 촉매의 오염물질을 제거하고 동시에 전극 구조를 다른 경우보다 개선한 것으로 추정할 수 있다[12]. 대조적으로 고전압에서 활성화 과정을 거친 연료전지는 활성화 작업 시 다른 경우에 비해 더 적은 전기화학 반응으로 인해 충분한 촉매 개선과 전극 구조 개선이 이루어지지 않아 Activation Resistance가 가장 높게 측정된 것을 알 수 있다.
또한, Figs. 4(b)와 4(c)를 통해 Activation Resistance의 변화가 Ohmic Resistance보다 더 뚜렷한 것을 확인할 수 있다. 이를 통해 활성화 과정은 Ohmic Resistance 개선과 Activation Resistance 개선 중 후자가 좀 더 지배적으로 이루어지는 것을 확인할 수 있다.
4. 결론
본 연구의 목적은 고분자 전해질 연료전지의 활성화 작업 시전압 범위를 제어하면서 해당 활성화 방법의 영향성을 살펴보았다. 이를 통해 다음과 같은 사실을 알 수 있었다.
1) 전압 범위에 따른 활성화 영향은 0.3-0.6 V에서 진행한 것이 가장 높은 성능을 출력한다.
2) 전압 영역에서는 더 많은 전자가 생성되고 이로 인해 더 많은 물이 생성되어 나피온의 수화와 동시에 전기화학 반응을 통해 촉매의 오염물질과 전극 구조가 개선되어 더 효과적인 활성화 영향을 가져온다.
3) 고전압 영역에서는 전기화학 반응이 덜 이루어지고 적은 물로 인해 충분한 나피온의 수화가 이루어지지 않고 전극 환경을 개선하는데 부족하다.
4) 고전압 영역에서는 부족한 활성화 과정으로 인해 오히려 연료전지에 부하를 가져온다.
Acknowledgments
이 논문은 2023년도 정부(산업통상자원부)의 재원으로 한국산업기술진흥원의 지원을 받아 수행된 연구임(P0017120, 2023년 산업혁신인재성장지원사업). 또한, 이 논문은 2023년도 정부(산업통상자원부)의 재원으로 한국에너지기술평가원의 지원을 받아 수행된 연구임(20213030030260, 항공용 모빌리티를 위한 연료전지 경량화 기술 개발). 또한, 이 논문은 정부(과학기술정보통신부)의 재원으로 한국연구재단의 지원을 받아 수행된 연구임(No. RS-2023-00213741).
REFERENCES
- Ryan, O., Cha, S.-W., Colella, W., Prinz, F., (2016), Fuel cell fundamentals, 3rd edition, Wiley.
-
Altaf, F., Batool, R., Gill, R., Rehman, Z. U., Majeed, H., Ahmad, A., Shafiq, M., Dastan, D., Abbas, G., Jacob, K., (2021), Synthesis and electrochemical investigations of ABPBI grafted montmorillonite based polymer electrolyte membranes for PEMFC applications, Renewable Energy, 164, 709-728.
[https://doi.org/10.1016/j.renene.2020.09.104]
-
Chu, D., Jiang, R., (1999), Performance of polymer electrolyte membrane fuel cell (PEMFC) stacks: Part I. Evaluation and simulation of an air-breathing PEMFC stack, Journal of Power Sources, 83(1-2), 128-133.
[https://doi.org/10.1016/S0378-7753(99)00285-2]
-
Zhang, X., Ni, M., Wang, J., Yang, L., Mao, X., Su, S., Yang, Z., Chen, J., (2020), Configuration design and parametric optimum selection of a self-supporting PEMFC, Energy Conversion and Management, 225, 113391.
[https://doi.org/10.1016/j.enconman.2020.113391]
-
Dafalla, A. M., Jiang, F., (2018), Stresses and their impacts on proton exchange membrane fuel cells: A review, International Journal of Hydrogen Energy, 43(4), 2327-2348.
[https://doi.org/10.1016/j.ijhydene.2017.12.033]
-
Santarelli, M., Torchio, M., (2007), Experimental analysis of the effects of the operating variables on the performance of a single PEMFC, Energy Conversion and Management, 48(1), 40-51.
[https://doi.org/10.1016/j.enconman.2006.05.013]
-
Gencoglu, M. T., Ural, Z., (2009), Design of a PEM fuel cell system for residential application, International Journal of Hydrogen Energy, 34(12), 5242-5248.
[https://doi.org/10.1016/j.ijhydene.2008.09.038]
-
Kuwertz, R., Kirstein, C., Turek, T., Kunz, U., (2016), Influence of acid pretreatment on ionic conductivity of Nafion® membranes, Journal of Membrane Science, 500, 225-235.
[https://doi.org/10.1016/j.memsci.2015.11.022]
-
Karimi, M. B., Mohammadi, F., Hooshyari, K., (2019), Recent approaches to improve Nafion performance for fuel cell applications: A review, International Journal of Hydrogen Energy, 44(54), 28919-28938.
[https://doi.org/10.1016/j.ijhydene.2019.09.096]
-
Liu, L., Chen, W., Li, Y., (2016), An overview of the proton conductivity of nafion membranes through a statistical analysis, Journal of Membrane Science, 504, 1-9.
[https://doi.org/10.1016/j.memsci.2015.12.065]
-
Ramani, V., Kunz, H., Fenton, J., (2004), Investigation of Nafion®/HPA composite membranes for high temperature/low relative humidity PEMFC operation, Journal of Membrane Science, 232(1-2), 31-44.
[https://doi.org/10.1016/j.memsci.2003.11.016]
-
Pei, P., Fu, X., Zhu, Z., Ren, P., Chen, D., (2022), Activation of polymer electrolyte membrane fuel cells: Mechanisms, procedures, and evaluation, International Journal of Hydrogen Energy, 47(59), 24897-24915.
[https://doi.org/10.1016/j.ijhydene.2022.05.228]
-
Zhiani, M., Majidi, S., Silva, V. B., Gharibi, H., (2016), Comparison of the performance and EIS (electrochemical impedance spectroscopy) response of an activated PEMFC (proton exchange membrane fuel cell) under low and high thermal and pressure stresses, Energy, 97, 560-567.
[https://doi.org/10.1016/j.energy.2015.12.058]
-
Qi, Z., Kaufman, A., (2002), Enhancement of PEM fuel cell performance by steaming or boiling the electrode, Journal of Power Sources, 109(1), 227-229.
[https://doi.org/10.1016/S0378-7753(02)00060-5]
-
Silva, V. B., Rouboa, A., (2012), Hydrogen-fed PEMFC: Overvoltage analysis during an activation procedure, Journal of Electroanalytical Chemistry, 671, 58-66.
[https://doi.org/10.1016/j.jelechem.2012.02.013]
-
Yuan, X.-Z., Sun, J. C., Wang, H., Li, H., (2012), Accelerated conditioning for a proton exchange membrane fuel cell, Journal of Power Sources, 205, 340-344.
[https://doi.org/10.1016/j.jpowsour.2012.01.039]
-
Zhiani, M., Majidi, S., (2013), Effect of MEA conditioning on PEMFC performance and EIS response under steady state condition, International Journal of Hydrogen Energy, 38(23), 9819-9825.
[https://doi.org/10.1016/j.ijhydene.2013.05.072]
-
Bezerra, C. W., Zhang, L., Liu, H., Lee, K., Marques, A. L., Marques, E. P., Wang, H., Zhang, J., (2007), A review of heat-treatment effects on activity and stability of PEM fuel cell catalysts for oxygen reduction reaction, Journal of Power Sources, 173(2), 891-908.
[https://doi.org/10.1016/j.jpowsour.2007.08.028]
M.S. candidate in the Department of Mechanical Engineering, Dankook University. His research interests are characterization of polymer electrolyte membrane fuel cells and thin films.
E-mail: dexasd916@naver.com
M.S. candidate in the Department of Mechanical Engineering, Dankook University. His research interest is solid oxide fuel cell and characterization of polymer electrolyte membrane fuel cells.
E-mail: dku72220126@dankook.ac.kr
B.S. candidate in the Department of Mechanical Engineering, Dankook University. His research interests are low-temperature solid oxide fuel cell and characterization of polymer electrolyte membrane fuel cells.
E-mail: 32184031@dankook.ac.kr
M.S. candidate in the Department of Mechanical Engineering, Dankook University. His research interest are computational fluid dynamics and characterization of polymer electrolyte membrane fuel cells.
E-mail: d72220125@dankook.ac.kr
M.S. candidate in the Department of Mechanical Engineering, Dankook University. Her research interest is characterization of polymer electrolyte membrane fuel cells.
E-mail: dpfla1262@dankook.ac.kr
M.S. candidate in the Department of Mechanical Engineering, Dankook University. His research interest are solid oxide fuel cells and characterization of polymer electrolyte membrane fuel cells.
E-mail: dudwh94@naver.com
M.S. candidate in the Department of Mechanical Engineering, Dankook University. His research interest are injection molding and characterization of polymer electrolyte membrane fuel cells.
E-mail: tmdgur3583@naver.com
M.S. candidate in the Department of Mechanical Engineering, Dankook University. Her research interest are plasma-enhanced atomic layer deposition and low-temperature solid oxide fuel cell.
E-mail: shomig28@gmail.com
B.S. candidate in the Department of Mechanical Engineering, Dankook University. Her research interest are injection molding and characterization of polymer electrolyte membrane fuel cells.
E-mail: rmaekgp99@naver.com
Assistant professor in the Department of Mechanical Engineering, Dankook University. He received his Ph.D. in Mechanical and Aerospace Engineering from Seoul National University. His research interest is fuel cells, thin films, and semiconducting processes
E-mail: guyoungcho@dankook.ac.kr