
반사형 항공우주 광학계 환경변화에 따른 이미지 품질 예측
Copyright © The Korean Society for Precision Engineering
This is an Open-Access article distributed under the terms of the Creative Commons Attribution Non-Commercial License (http://creativecommons.org/licenses/by-nc/3.0) which permits unrestricted non-commercial use, distribution, and reproduction in any medium, provided the original work is properly cited.
Abstract
The use of reflective optical systems is essential to acquiring high-resolution image quality in aerospace applications that observe distant objects. The geometric shapes of large-aperture reflective optical systems can be deformed depending on various operating and space environments, which deformation consequently affects optical performance. In this study, we predict the image quality of a reflective aerospace optical system according to various environmental changes. In particular, the shape deformation due to vibration and heat generated from the launch vehicle was mainly observed, and the effect on gravity was also considered. The variations of image quality, such as Modulation Transfer Function (MTF) and wave-front error (WFE), were also observed by importing the deformed shapes into the optical simulation tool. This study is intended to provide approaches to reduce the cost and lead time to develop aerospace optical systems.
Keywords:
Reflective optical systems, Environment simulation, Deformation, Image quality, Optical mirror키워드:
반사형 광학 시스템, 환경 시뮬레이션, 변형, 이미지 품질, 광학 거울1. 서론
항공우주 관측시스템은 원거리에 위치한 물체를 고해상도 이미지로 획득하기 위하여 색수차가 작고 집광력이 높은 대구경 반사형 광학계를 주로 사용한다[1,2]. 대구경 반사형 광학계는 원거리에서 전달되는 빛의 정보를 효율적으로 집광할 수 있다는 장점이 있지만, 큰 형상으로 인해 외부 환경에 의해 변형이 발생할 수 있다. 반사형 광학 부품의 형상 변형은 이미지 품질에 영향을 줄 수 있으며, 각 광학 부품들의 정렬 또한 외부 환경에 의해 변형되어 이미지 품질을 저해시키는 원인이 될 수 있다. 항공우주 관측용 광학계는 지상에서 우주로 이동하는 동안 온도, 발사체의 진동, 중력 및 대기 압력 등의 외부 환경에 의해 변형이 될 수 있으며, 이러한 환경 변화를 극복하기 위하여 다양한 소재와 구조를 적용한 연구들이 진행되었다[3-5]. 반사형 거울로 주로 상용되는 소재에는 탄화규소(Silicon Carbide, SiC), 알루미늄 합금(Aluminum Alloy), 제로듀어(Zerodur), 베릴륨(Beryllium, Be) 등이 있으며, 각 소재의 기계적 특성 및 비용을 고려하여 선정한다[6]. 제임스 웹 우주망원경(James Webb Space Telescope)의 경우 무게 대비 강성이 높고 열 안정성이 뛰어난 베릴륨 소재를 이용하여 반사형 거울을 제작하였다[7]. 최근 제임스 웹 우주망원경 후속으로 개발되고 있는 외계 행성 관측 망원경(Habitable Exoplanet Observatory, HabEx)은 제로듀어 소재를 적용할 것으로 소개되고 있다[8]. 탄화규소는 다른 소재들과 비교하여 매우 뛰어난 열 및 기계적 안정성을 가지고 있으나 비용적인 측면과 치수 안정성을 고려하여 반사형 거울 소재로 적용된다[9,10].
발사체가 우주로 이동하는 동안 다양한 환경변화를 경험하게 되는데, 우주와 동일한 환경을 구현하는 것은 매우 어렵고 많은 비용이 소모되기 때문에 환경 수치 시뮬레이션을 통하여 우주항공 광학 시스템의 변형을 예측한다[11-13]. 헤라(HERA) 탐사선에 적용된 반사형 광학계의 변형을 분석하는 연구가 최근 소개되었으며, 이 연구에서는 제로듀어 거울 및 이를 지지 하는 티타늄 소재의 양각대가 온도에 따라서 변화되는 정도를 관측하였다[14]. 열팽창을 최소화하기 위해 거울과 하우징을 동일한 소재로 적용한 광학계의 열 환경 시뮬레이션을 진행한 연구도 소개되었으며, 형상 변화에 따른 광학적 특성 변화도 분석을 하였다[15]. 하지만 대부분의 연구에서 온도에 대한 변형 시뮬레이션에 한정되어 있어, 탑재체가 우주를 이동하는 동안 경험할 수 있는 다양한 외부 환경적 요인에 따른 광학적 특성 변화에 대한 연구들이 부족한 상황이다.
본 논문에서는 다양한 환경 변화에 따른 반사형 항공우주 광학계의 이미지 품질을 예측하는 연구를 소개한다. 반사형 광학계 이미지 품질 예측을 위하여 광학계 형상 및 광학 설계를 수행하고 운용 및 외부 환경 조건에 대하여 정의하였다. 그리고, 발사체에서 발생하는 기계적 진동, 열 및 중력의 환경 변화에 따라서 반사형 광학계가 받는 응력과 변위를 분석하였다(Fig. 1). 광학 수치해석 프로그램(Ansys Zemax)을 통하여 변형된 형상에 따른 변조 전달 함수(MTF) 및 파면 오차(Wavefront Error, WFE)와 같은 광학적 성능 변화를 분석하였다.
2. 반사형 광학계 변형 및 이미지 품질 예측 방법
2.1 운용 우주환경 조건 정의
국제항공연맹(Federation Aeronautique Internationale, FAI)에 따르면 고도 100 km 상공이 지구의 대기권과 우주 공간을 구분하는 경계로 정의하며, 이를 카르만 선(Kármán Line)이라고 불린다. 카르만 선 이상에서는 대기의 저항이 매우 작은 상태에서 비행이 가능하기 때문에, 본 연구에서는 고도 100 km에서의 반사형 항공우주 광학계 환경변화를 예측하였다. 고도 100 km에서의 중력가속도는 9.499 m/s2 이며, 온도 184 K(-89.15oC), 밀도 5.08 × 10-07 kg/m3, 그리고 압력 2.81 × 10-03 Pa의 환경조건을 갖는다[16]. 대부분의 우주비행선은 대기권을 벗어나기 위하여 고도 100 km에서 8 마하 이상의 속도로 움직이나, 높은 고도로 인하여 공기 밀도가 낮아 광학계가 위치한 우주비행선 앞부분의 열유속(Heat Flux)은 0 kW/m2 에 수렴한다[17]. 고도 100 km에서 공기 저항에 의한 가열은 매우 작기 때문에, 엔진 추진체에서 발생하는 열 및 외부 온도차이에 의한 광학계 열 변형을 분석하였다. 또한, 우주비행선이 비행을 하며 발생할 수 있는 진동 조건에 대하여 분석을 하였으며, 그에 따른 광학계의 변형 및 광학 성능 변화를 시뮬레이션 하였다.
2.2 반사형 광학계 변형 및 광학 성능 예측 방법
우주 환경에서의 반사형 광학계 변형 예측을 수행하기 위하여 다중물리 해석 프로그램 Solidworks Simulation 및 광선 추적 프로그램 Ansys Zemax 를 통하여 물리적 변형과 이미지 품질을 예측하였다(Fig. 2). 해석하고자 하는 반사형 광학계 모델의 크기는 280 × 280 × 313 mm3 이며 주경(Primary Mirror), 부경(Secondary Mirror), 빛가림 경통(Sun Shade), 양각대(Tripod), 지지대(Support) 및 카메라 센서로 구성된다. 반사형 광학계의 재료는 SiC, Al6061, Al7075, Ti-6-4 등이 적용되었다(Table 1). 변형 해석을 위하여 생성된 메쉬(Mesh)는 총 절점 수 128,808개, 총 요소 수 69,982개이며, 주로 관측하고자 하는 영역인 미러 광학계 표면의 메쉬는 기타 기구물 보다 더 세밀화하여 구성하였다. 반사형 광학계의 하부 사각 플레이트가 우주비행선 내부에 고정이 되어있다는 구속조건을 설정하여 광학계의 변형 정도를 분석 하였다. 우주비행선의 엔진 추진체에서 발생하는 열이 열전달을 통하여 광학계 변형에 미치는 영향을 예측하였고, 우주비행선에서 발생할 수 있는 임의 진동을 정의하여 분석을 진행하였다. 반사형 광학계의 물리적 변형 데이터의 공간 정보는 Zemax STAR Module을 통하여 광학 설계 및 분석소프트웨어에 연동을 시켰다. 미러 광학계의 면의 변형 정보를 광학 설계형상 데이터에 매칭을 시켰으며 광학 소프트웨어를 통하여 MTF 및 WFE와 같은 광학 성능 변화를 분석하였다.
3. 반사형 광학계 변형 및 이미지 품질 예측 결과
3.1 반사형 광학계 변형 예측 결과
관측 환경인 100 km의 고도에서는 중력가속도 9.499 m/s2로 지상보다는 다소 낮지만, 광학계 방향에 따른 자중에 의한 변형이 발생할 수 있어 변형 정도를 관측 하였다(Fig. 3). 발사체가 가속을 위해 수직 방향에서 수평으로 자세를 조정하였을 때를 가정하였으며, 광학계와 수평 방향으로 중력이 작용하였을 때 광학계의 최대 응력 0.58 MPa, 최대 변위는 0.125 μm로 예측되었다. 광학계와 중력이 수직을 이루었을 때는 최대 응력 0.2501 MPa, 최대 변위 0.172 μm의 결과를 보였으며 이는 중력에 의한 변형은 매우 작다는 것을 나타낸다.
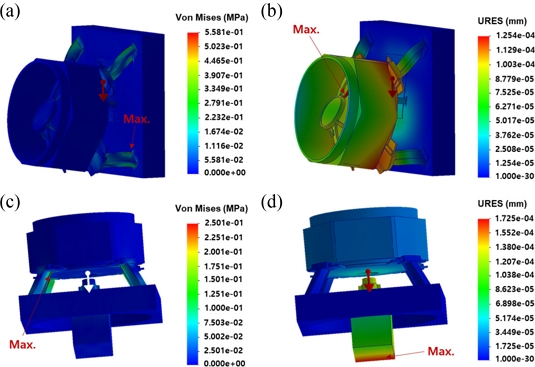
Deformation results of the reflective optical system due to gravity. (a) Stress, (b) displacement results when the force of gravity is applied in a parallel direction to the mirror, (c) Stress, and (d) displacement when gravity is applied in the direction perpendicular to the mirror
열에 의한 광학계 변형은 다양한 온도 조건에서 정상상태를 가정하여 분석을 진행하였다(Fig. 4). 초기 20oC 대비 30-70oC 까지 정상상태에서의 온도에 대한 변형량 및 변위를 분석하였으며 X, Y, Z 각 변위의 합을 통하여 최대 변위를 계산하였다. 정상상태 70oC 가정조건에서는 X축 최대 변위 243 μm, Y축 최대변위 143 μm, 그리고 Z축 최대 변위 164 μm를 나타내었고 총합 변위 270 μm의 변위를 보였다. 최대 변위가 발생하는 부분은 경통에서 주로 변형이 이루어져 광학 면에서의 최대 변위를 관측한 결과 120 μm로 분석되었다. 이 때 최대 변형률은 2.31 × 10-03으로 나타났으며, 정상상태 온도가 낮을수록 변위가 감소하는 결과가 나타났다. 열변형 분석 결과 고정되어 있는 면에서 멀어질수록 변위가 크게 나타나는 것을 확인하였으며, 광학 미러보다 경통 부분이 더 크게 변형이 일어나는 것을 확인하였다.
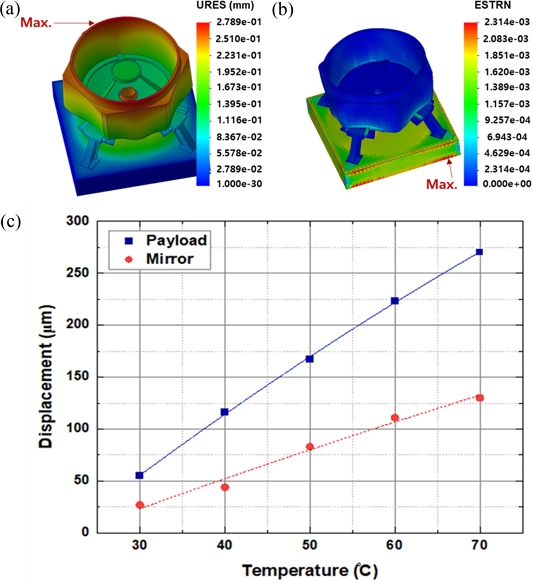
Thermal deformation results of the reflective optical system. (a) Displacement, (b) strain at 70oC steady-state temperature, and (c) Maximum displacement graph of the reflective optical system according to steady-state temperature
설계된 반사형 광학계의 고유진동수 해석을 통하여 모드 현상, 공진 및 질량 기여도에 대한 정보를 분석하였다(Fig. 5). 총 20개의 모드 번호에 따른 고유진동수를 분석하였고, 얼마나 많은 질량이 구조 안에서 특정 방향으로 움직이는지를 파악하기 위하여 모드에 따른 질량 분배를 정규화 하여 분석 하였다. 분석 결과 X축에서의 질량 분배가 평균 0.212로 가장 높았으며, Y축 0.0172 및 Z축 0.0176의 질량 분배 결과를 나타내었다. 특히 모드 10번에서의 X축 질량 분배가 가장 높게 분석이 되었으며, 이를 통하여 진동에 지배적인 모드를 식별 가능하다.
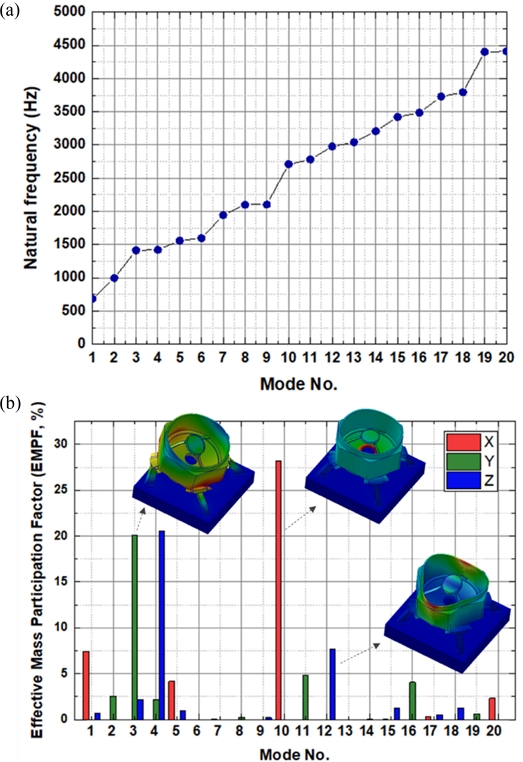
Natural frequency analysis of the reflective optical system. (a) Natural frequency and (b) Effective mass participation factor results according to different modes
발사체 엔진 및 공기 마찰에 의해 불규칙 임의 진동이 발생할 수 있어, MIL-STD-810F (Method 514.5) 진동 규격을 이용하여 주파수 스펙트럼 분석을 진행하였다(Fig. 6). 불규칙 임의 진동에 대하여 RMS (Root Mean Square) 정규분포 및 감쇠비(Damping Ratio)에 따른 최대 변위를 관측하였으며, 진동에 의한 변위 최소화를 위하여 주요 변형 위치를 분석하였다. 감쇠비가 0.01의 경우 최대 변위가 31.6 μm로 분석되었으며, 감쇠비가 0.02로 높아지면 최대 변위가 22.8 μm까지 감소한다. 또한 감쇠비가 0.04까지 높아지면 최대 변위는 16.1 μm 수준까지 낮아지며, 주로 경통을 지지하는 지지대 부분에서 변형이 발생하는 것을 관측하였다. 지지대 지지 방향에 추가적인 감쇠 장치를 보완하면 감쇠비를 증가시켜 진동에 의한 영향을 감소 시킬 수 있을 것으로 판단된다.
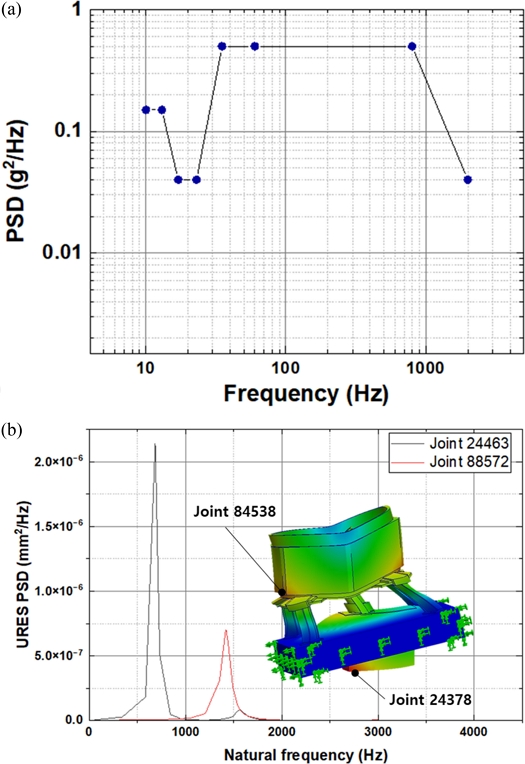
Deformation results according to random vibration. (a) The Shipboard random vibration exposure (MIL-STD 810F, Method 514.5) and (b) Power spectral density (PSD) frequency displacement response according to natural frequency
반사형 광학계가 우주로 발사되기 전과 추진체와 분리되는 과정 중에 물리적 충격에 의한 변형이 추가로 발생할 수 있어, MIL-STD-810H 방법을 통하여 충격에 의한 진동 및 변형을 분석하였다(Fig. 7). 충격에 대한 조건은 최대 20 g의 힘이 0.011 sec 동안 선형적으로 증가하다가 급격히 힘이 제거되었을 때를 가정하여 해석을 수행하였다. 측면 방향과 평행 방향에서 충격이 발생하였을 때를 분석한 결과, 측면 방향에서 충격이 가해졌을 때는 0.011초에서 미러 광학계 최대 변위 1.5 μm 가 발생하였다. 반면 평행 방향 충격에서는 최대 변위 0.04 μm로 미러 광학계에서는 변형이 거의 일어나지 않는 것을 확인하였다. 반사형 광학계 변형 예측 결과 열에 의한 형상 변형이 가장 크게 나타난다는 것을 확인 하였으며, 중력이나 충격에 의한 변형은 매우 작다는 것을 확인 하였다.
3.2 반사형 광학계 이미지 품질 예측 결과
반사형 광학계 변형 예측 결과 형상을 Ansys Zemax OpticStudio의 Star Module을 통하여 광학 설계면에 매칭시켜 이미지 품질을 분석하였다. 변형 해석 형상의 포인트들을 텍스트파일로 추출한 후 Zemax에서 형상을 불러들여 축을 정렬시킨 후 해당 거울 면에 매칭시켜 정보를 전달하였다. 이미지 품질을 분석하기 위하여 변형 해석 전/후의 WFE 값과 MTF 두 수치를 비교하였는데, WFE는 형성된 파면의 가장 높은 피크(Peak)와 골(Valley)을 분석하는 Peak to Valley (PV) 값과 전체 오차의 평균을 계산하는 RMS 값을 관측하였다. MTF는 공간 주파수의 샘플 대비(Contrast)를 나타내는 수치로 대비 0.5일 때의 공간 주파수 값인 MTF50 수치를 통하여 분석하였다.
변형 해석 전의 반사형 광학계의 WFE는 PV 0.0138 λ, RMS 0.0035 λ로 매우 형상이 고르고 및 정렬이 잘된 결과를 나타내었다. 하지만 열 변형에 대한 주경 및 부경의 해석 형상을 기존 광학 설계 면에 매칭시켜 분석을 한 결과 PV 5.043 λ, RMS 1.340 λ로 WFE의 수치가 변화하는 것을 확인하였다(Fig. 8). 이미지의 해상도를 평가하는 지표인 MTF결과에서도 변형 해석 전 중심 기준 MTF50은 16.08 lp/mm의 결과를 보였지만, 열 변형 해석 후 중심 기준 MTF50의 수치는 4.97 lp/mm로 저해가 되는 것을 확인하였다(Fig. 9). 진동에 의해 발생한 미러 변형의 이미지 품질 영향을 분석한 결과 PV 0.485 λ, RMS 0.1338 λ로 WFE 수치가 변화되는 것을 확인하였다. 또한 중심 기준 MTF50 수치는 15.91 lp/mm로 변화가 크게 나타나지 않는 것을 확인하였다. 이러한 결과들을 통하여 반사형 광학계는 열 변형에 의한 이미지 품질 저하가 가장 크게 나타나는 것을 확인 할 수 있으며, 이러한 품질 저하를 방지하기 위하여 열 변형을 고려한 최적화 설계가 필요하다는 것을 분석 결과가 나타낸다.
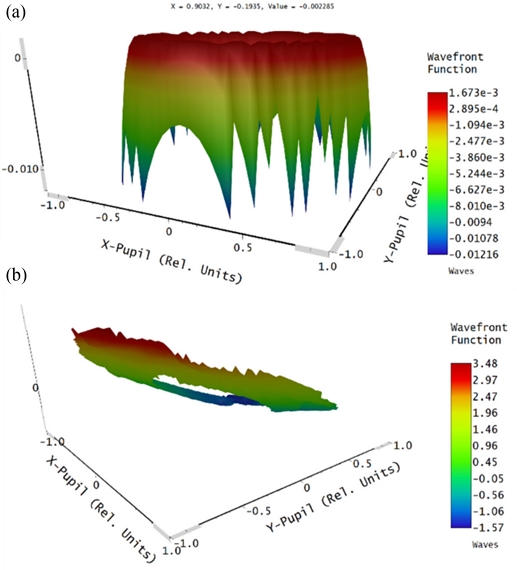
Wave-front error analysis. Wave-front error analysis results according to (a) before and (b) after the deformation by thermal shock
4. 결론
본 논문에서는 우주 환경변화에 따른 반사형 광학계의 형상 변형과 광학적 성능 변화를 예측하는 연구를 소개하였다. 본 연구에서는 지구의 대기권과 우주 사이의 경계를 정의하는 고도 100 km의 카르만 선의 온도 및 중력 요인을 분석하고, 추진체의 진동 및 충격을 입력시켜 반사형 광학계에 미치는 영향을 분석하였다. 시뮬레이션 결과 자중에 의한 영향은 무시할 정도로 작았으며, 열 전달에 의한 변형이 가장 크게 나타났다. 광학적 성능 변화 또한 열 전달에 의한 변형에 의해 가장 크게 저해되었으며, 파면 오차에 대한 RMS값이 1.340 λ까지 증가하는 결과를 보였다. 진동에 의한 변형은 열에 의한 영향보다 작게 분석이 되었으며, 진동 따른 파면 오차 RMS 값이 0.1338 λ로 분석되었고 MTF 수치 15.91 lp/mm로 크게 변동되지 않는 것을 확인하였다. 이러한 접근 방법을 통하여 광학적 성능 변화를 최소화하는 디자인 설계 연구에 직접적인 기여를 할 수 있으며, 제작 허용오차 범위 설정 및 경제적인 설계에도 도움을 줄 것으로 예상된다.
Acknowledgments
This work was supported by the Agency for Defense Development Grant Funded by the Korean Government (No. UI230005SD).
REFERENCES
-
Kim, K., Bae, J.-H., Park, J., (2023), Reflective optical systems for aerospace applications, Journal of the Korean Society for Precision Engineering, 40(11), 899-906.
[https://doi.org/10.7736/JKSPE.023.080]
-
Lightsey, P. A., Atkinson, C. B., Clampin, M. C., Feinberg, L. D., (2012), James webb space telescope: large deployable cryogenic telescope in space, Optical Engineering, 51(1), 011003-011003.
[https://doi.org/10.1117/1.OE.51.1.011003]
-
Zhang, C., Li, Z., (2022), A review of lightweight design for space mirror core structure: tradition and future, Machines, 10(11), 1066.
[https://doi.org/10.3390/machines10111066]
-
Wu, J., (2020), Design of high-lightweight space mirror component based on automatic optimization, Journal of Physics: Conference Series, 012023.
[https://doi.org/10.1088/1742-6596/1605/1/012023]
-
Jalluri, T. D. P. V., Rao, B. V., Rudraswamy, B., Venkateswaran, R., Sriram, K., (2023), Optical polishing and characterization of chemical vapour deposited silicon carbide mirrors for space applications, Journal of Optics, 52(3), 969-983.
[https://doi.org/10.1007/s12596-022-00925-w]
-
Wu, H.-Y., Chang, W.-C., Huang, P.-H., Huang, Y.-K., (2019), New candidate substrate materials for space mirror optics, Proceedings of the Material Technologies and Applications to Optics, Structures, Components, and Sub-Systems IV, 157-161.
[https://doi.org/10.1117/12.2530689]
-
Gardner, J. P., Mather, J. C., Clampin, M., Doyon, R., Greenhouse, M. A., Hammel, H. B., Hutchings, J. B., Jakobsen, P., Lilly, S. J., Long, K. S., Lunine, J. I., Mccaughrean, M. J., Mountain, M., Nella, J., Rieke, G. H., Rieke, M. J., Rix, H.-W., Smith, E. P., Sonneborn, G., Stiavelli, M., Stockman, H. S., Windhorst, R. A., Wright, G. S., (2006), The james webb space telescope, Space Science Reviews, 123, 485-606.
[https://doi.org/10.1007/s11214-006-8315-7]
-
Philip Stahl, H., Kuan, G., Arnold, W. R., Brooks, T., Brent Knight, J., Martin, S., (2020), Habitable-zone exoplanet observatory baseline 4-m telescope: systems-engineering design process and predicted structural thermal optical performance, Journal of Astronomical Telescopes, Instruments, and Systems, 6(3), 034004.
[https://doi.org/10.1117/1.JATIS.6.3.034004]
-
Hull, T., Westerhoff, T., Weidmann, G., (2015), Selection considerations between ZERODUR® and silicon carbide for dimensionally-stable spaceborne optical telescopes in two-earth-orbits, Proceedings of the SPIE Optical Engineering + Applications 2015, 108-117.
[https://doi.org/10.1117/12.2188770]
-
Kasunic, K. J., Aikens, D., Szwabowski, D., Ragan, C., Tinker, F., (2017), Technical and cost advantages of silicon carbide telescopes for small-satellite imaging applications, Proceedings of the Earth Observing Systems XXII, 10402, 110-126.
[https://doi.org/10.1117/12.2274048]
-
Jeong, S., Nam, J.-H., Ahn, K.-B., Park, I. H., Kim, S. W., Lee, J., Lim, H., Brandt, S., Budtz-Jørgensen, C., Castro-Tirado, A. J., Chen, P., Cho, M. H., Choi, J.-N., Grossan, B., Huang, M.-H. A., Jung, A., Kim, J. E., Kim, M. B., Kim, Y.-W., Linder, E. V., Min, K. W., Na, G., Panasyuk, M. I., Ripa, J., Reglero, V., Smoot, G., Suh, J., Svertilov, S. I., Vedenkin, N., Yashin, I. V., (2013), Slewing mirror telescope optics for the early observation of UV/optical photons from gamma-ray bursts, Optics Express, 21(2), 2263-2278.
[https://doi.org/10.1364/OE.21.002263]
-
Tan, S., Zhang, X., Wang, L., Wu, H., Fu, Q., Yan, L., Hu, M., (2022), Equivalent modeling and verification of a high-steepness and lightweight elliptical aluminum mirror, Applied Sciences, 12(18), 9091.
[https://doi.org/10.3390/app12189091]
-
Lee, J.-H., Lee, C.-W., Kim, Y.-M., Kim, J.-W., (2009), Optomechanical design of a compact imaging spectrometer for a microsatellite STSAT3, Journal of the Optical Society of Korea, 13(2), 193-200.
[https://doi.org/10.3807/JOSK.2009.13.2.193]
-
Dias, N. G., Gordo, P., Onderwater, H., Melicio, R., Amorim, A., (2022), Analysis on the isostatic bipod mounts for the HERA mission LIDAR, Applied Sciences, 2022, 12(7), 3497.
[https://doi.org/10.3390/app12073497]
-
Peschel, T., Damm, C., Beier, M., Gebhardt, A., Risse, S., Walter, I., Sebastian, I., Krutz, D., (2017), Design of an imaging spectrometer for earth observation using freeform mirrors, Proceedings of the International Conference on Space Optics-ICSO 2016, 10562.
[https://doi.org/10.1117/12.2296156]
- National Oceanic and Atmospheric Administration, (1976), US standard atmosphere, (Report No. NOAA-S/T-76-1562), https://ntrs.nasa.gov/citations/19770009539
-
Laureti, M., Karl, S., (2022), Aerothermal databases and load predictions for retro propulsion-assisted launch vehicles (RETALT), CEAS Space Journal, 14(3), 501-515.
[https://doi.org/10.1007/s12567-021-00413-0]
received his Ph.D. degree in the department of Bio and Brain Engineering from Korea Advanced Institute of Science and Technology (KAIST), in 2021. Now, he is a senior researcher at Korea Photonics Technology Institute(KOPTI). His research interests include optical module design and evaluation for arrayed camera systems.
E-mail: kisookim@kopti.re.kr
received his Ph.D. degree in the School of Mechanical Engineering from Chung-Ang University, in 2018. Now, he is a senior researcher at Agency for Defense Development(ADD). His research interests include EO/IR systems.
E-mail: ghunb16@add.re.kr
received his Ph.D. degree in the department of Mechatronics Engineering from Gwangju Institute of Science and Technology (GIST), in 2009. Now, he is a director of Intelligent Optical Module Research Center at Korea Photonics Technology Institute(KOPTI). His research interests include optical system design for laser and imaging optic system.
E-mail: jb.park@kopti.re.kr