
주파수 변조 흡수 분광법 기반 극미량 누설 가스 원격 검출 기술
Copyright © The Korean Society for Precision Engineering
This is an Open-Access article distributed under the terms of the Creative Commons Attribution Non-Commercial License (http://creativecommons.org/licenses/by-nc/3.0) which permits unrestricted non-commercial use, distribution, and reproduction in any medium, provided the original work is properly cited.
Abstract
The LIDAR principle is used in a variety of fields, including large-scale pipeline facility management, industrial disaster safety control, and atmospheric environmental monitoring, to employ the remote gas detection technique. In this study, we designed and implemented a remote detection method for N2O gas leaks using absorption spectroscopy based on frequency modulation of a Mid-IR quantum cascade laser (QCL) with a wavelength of 4.5 μm. We direct the frequency-modulated beam, locked to a single absorption line of N2O, to a leak hole on a target surface within a range of approximately 50 m. For area scanning around the leak point, we use a galvano scanner to deflect the probe beam. The back-scattered beam from the diffuse target surface is then collected by a Cassegrain telescope with a diameter of 300 mm and detected by an InSb photo-detector with high photon sensitivity. To process the detected signal, we utilize fundamental and second harmonic detection with a lock-in amplifier, resulting in a relative gas concentration expressed as the second harmonic signal normalized by the fundamental signal. Our test results demonstrate that this proposed method can detect gas leaks as small as 0.005 sccm at a distance of 50 m.
Keywords:
Leak gas, Absorption spectroscopy, Frequency modulation, Remote detection키워드:
누설가스, 흡수 분광, 주파수 변조, 원격 검출1. 서론
LiDAR (Light Detection And Ranging)와 같이 빛을 이용한 원거리 대상에 대한 원격 탐지 기술은 지형 탐사, 대기 환경 모니터링, 온도 측정과 같은 다양한 분야에서 활용되고 있다[1-6]. 원리적으로 LiDAR 기술은 측정하고자 하는 대상에 빛을 조사하여 후방산란 되는 신호를 검출 및 분석함으로써 구현된다. 여기서 산란광의 크기가 매우 작을 경우, 산란광의 검출 효율을 높이기 위한 고출력 광원, 효율적인 수광부 설계, 고민감도 측정 기술이 반드시 필요하다. 이와 같은 LiDAR 기반 고민감도 측정은 산업 안전성 및 경제성 확보에 있어서 매우 중요한 과제인 원격 가스 누설 검출 분야에도 적용되어 왔다[7].
본 논문에서는 아산화질소(N2O) 극미량 누설 검출을 위한 중적외선 레이저 주파수 변조 흡수 분광 측정 기술을 제안하고, 이를 위한 고민감도 검출 시스템을 설계 및 구현하였다. 주파수 변조 흡수 분광법을 구현하기 위해서는 광원의 주파수 변조가 필수적이며, 이는 광원의 입력 전류 변조를 통해 이루어진다. 본 연구에서 사용한 레이저 광원의 특성상 광원의 전류 변조 과정에서 광원의 광량 변화는 필연적으로 발생한다. 이러한 주파수 변조에 따른 광량 변화 크기를 측정 및 보상하여 극미량 누설 가스 검출 민감도를 향상시키기 위해 변조 주파수뿐만 아니라 2차 조화파 성분도 동시에 획득하여 분석하였다. 이를 통해 최대 50 m의 측정 거리에서 누설 타깃 면 (Leakage Target Surface)의 영역을 스캔하여 0.005 sccm (Standard Cubic Centimeter per Minute)의 매우 높은 가스 검출 민감도를 구현할 수 있다.
2. 주파수 변조 흡수 분광법 기반 측정 원리
식(1)은 Fig. 1과 같이 빛이 매질을 통과할 때 매질 내에서의 흡수에 의한 투과 광량의 감쇠를 정량적으로 계산하기 위해 사용되는 비어-램버트 법칙(Beer-Lambert Law)을 나타낸다. Fig. 1에서 나타낸 바와 같이 식(1)에서 I0는 입사광의 세기, Idet는 투과광의 세기, λ는 빛의 파장, lint는 매질 내 빛의 전파 경로 길이, σ(λ)는 물질 고유 특성에 해당하는 흡수 단면(Absorption Cross Section, 단위: cm2), n은 단위 부피당 존재하는 가스 분자 수(단위: cm-3)를 의미한다. 예를 들어, 본 연구에서 검출용 누설가스로 사용된 아산화질소의 경우에는 파장 4.54 μm에서 흡수 단면인 σ값이 3 × 10-18cm2를 가진다[8]. 즉, 매질의 흡광도(Absorbance, A = ln(Idet/I0))는 매질을 통과하는 거리인 lint과 매질의 흡수 단면인 σ에 비례하는 형태로 간단히 표현할 수 있다[9,10].
(1) |
앞서 언급한 바와 같이 본 논문에서는 누설 검출용 가스로 아산화질소를 사용하였다. 이는 비활성, 비불연 가스로 실제로 안전상의 이유로 대형 천연가스 수송용 가스탱크 내부 용접 부위 누설 위치 검출을 위한 가스 중의 하나로 사용되고 있다. HITRAN 데이터베이스에 따르면, 아산화질소 가스는 2,223.5 cm-1 (파장으로는 약 4.498 μm) 파수 근처에서 강한 흡수 대역을 가지고 있고, 대기 중에서 대략 320 ppb 수준의 농도를 가지고 존재한다고 알려져 있다[11-13].
Fig. 2는 주파수 변조 방법을 이용한 흡수 분광에 의한 매질의 단일 흡수선에서의 투과광의 세기 변화 특성을 나타내는 그래프이다. 분홍색 그래프는 매질의 투과율(Transmittance)을 나타내는데, 실선과 점선은 각각 매질의 농도에 따라 흡수선 골의 깊이가 큰 경우와 상대적으로 작은 경우를 나타낸다. 파란색 그래프와 같이 특정 흡수선을 기준으로 변조 주파수 f, 변조 진폭 Δνmod를 가지는 사인파 형태로 주파수 변조를 수행하게 될 경우, 투과광은 변조 주파수의 2배에 해당하는 2f로 변조되어 나타나게 된다. 여기서, 흡수선 골의 깊이, 즉 매질의 농도에 비례하여 변조된 투과광 신호의 진폭은 달라지며, 이와 같은 진폭의 크기 비교를 통해 해당 가스 매질 농도의 상대적인 변화를 확인할 수 있다.
그러나, 이론적으로 주파수 변조에 의한 흡수 분광 신호에는 Fig. 2와 같이 매질의 흡광도에 의한 2f에 해당하는 주파수 성분 외에 레이저 자체의 광강도 변조 성분 또한 포함된다. Fig. 3은 가스 분자 흡수선 ν0를 중심으로 주파수 변조(fmod)를 위한 전류 변조에 의해 발생하는 레이저 광강도의 1차 및 2차 변조 성분과 흡수 분광에 의한 신호를 도식화하여 나타낸 것이다. 먼저, 레이저 자체 광강도 변조에 의한 신호는 식(2)와 같이 나타낼 수 있다. 식(2)에서 P0는 레이저 광원의 DC 출력, Δ1f와 Δ2f는 레이저 광강도에 발생하는 1차 및 2차 변조 주파수 성분 진폭의 DC 크기 대비 비율, ϕ1f와 ϕ2f는 1차 및 2차 변조 주파수 성분의 위상을 의미한다. 즉, 주파수 변조에 의해 발생하는 레이저 광강도 변화는 두 성분의 합으로 표현된다. 이때, 식(2)에서 부호가 음수인 이유는 주파수 변조 신호와 광량 변화 신호의 위상이 180도 차이 나기 때문이다. 이는 광원의 특성에 따라 달라질 수 있으므로, 실험적으로 확인할 수 있다. 반면, 매질의 흡수에 의한 변조 신호는 식(3)과 같이 나타낼 수 있다. 식(3)에서 A0는 기준 흡광도, Δabs는 흡수 분광 신호의 변조 진폭, ϕabs는 흡수에 의한 2차 변조 주파수 성분의 위상을 의미한다. 결과적으로 주파수 변조에 의해 획득하게 되는 최종적인 변조 신호(Vsig)는 레이저 광강도 변화를 나타내는 식(2)와 이와 같이 변화하는 광강도가 매질의 흡광도에 의해 변조되는 식(3)을 조합하여 식(4)와 같이 모델링할 수 있다.
(2) |
(3) |
(4) |
3. 측정 시스템 구성 및 테스트 결과
3.1 측정 시스템 구성
Fig. 4는 본 연구에서 제안된 주파수 변조 흡수 분광법을 이용한 누설 가스 검출 시스템의 광학 구성도를 나타낸다. 먼저, 광원으로는 아산화질소 가스 누설 측정을 위해 중심 파장 4.5 μm, 출력 200 mW를 가지는 파장 가변 중적외선 QCL(Quantum Cascade Laser) 광원이 사용되었다[14-16]. 광원으로부터 전파된 빛은 광단절기(Optical Isolator), 빔확장기(Beam Expander), 갈바노 스캐너(Galvano-scanner)를 통해 누설 벽면상의 특정 위치에 조사된다. 타깃 벽면은 실제 천연가스 수송용선박 가스 탱크 소재로 사용되는 인바(Invar)를 사용하여 제작 되었고, 타깃 벽면의 중심 위치에 미세한 구멍을 뚫어 미량의 가스가 누설되는 상황을 모사하였다. 이때, 누설되는 가스의 유량은 유량 측정제어기(Mass Flow Controller, MFC)를 사용하여 조절할 수 있도록 하였다. 타깃 벽면은 측정 시스템으로부터 50 m 떨어진 위치에 설치하였고, 타깃 벽면으로부터 후방 산란된 빛은 카세그레인(Cassegrain) 형태의 망원경과 고감도 InSb 광검출기로 이루어진 수광부 모듈을 통해 검출된다. 카세그레인 망원경은 구경이 300 mm이고, 부경에는 3축 미세 스캐너를 부착하여 타깃 벽면에 빛이 조사된 위치에 따라 부경의 광축 위치, 피치(Pitch), 요(Yaw) 움직임을 동기화시켜 구동함으로써 산란광 검출 효율을 최대화할 수 있도록 구성하였다. 광원의 주파수 변조는 함수 발생기에 의해 생성된 1 kHz의 정현파 신호를 광원 제어기의 전류 단자에 입력하여 구현된다. 이와 같은 주파수 변조 신호는 Figs. 2와 3에 나타낸 바와 같이 1차 성분(f)과 2차 조화파 성분(2f)에 해당하는 광량 변조 신호 크기를 검출하기 위한 락인앰프(Lock-in Amplifier)의 입력으로도 사용된다. 본 측정 시스템에서는 산란광뿐만 아니라 주파수 변조에 의해 광원 자체에 발생하는 f 및 2f 성분 크기를 측정하여 광량 변동 영향을 보상해 주기 위한 목적으로 총 2대의 락인앰프가 사용되었다. 또한, 중적외선 대역의 빛은 육안으로 확인하기 어렵기 때문에 광학 정렬을 용이하게 하고, 중적외선 빛의 조사 위치를 정확히 확인하기 위해 가시광 대역 532 nm 파장의 정렬용 레이저를 별도로 동일 광경로에 설치하였다. Figs. 5는 4와 동일하게 실제로 구현 및 설치된 실험 장치 시스템을 나타낸다. 측정 시스템을 구성하는 광학계 전체는 광학 테이블 상에 설치되었고, 건물 지하에 50 m의 실험 공간을 확보하였다.
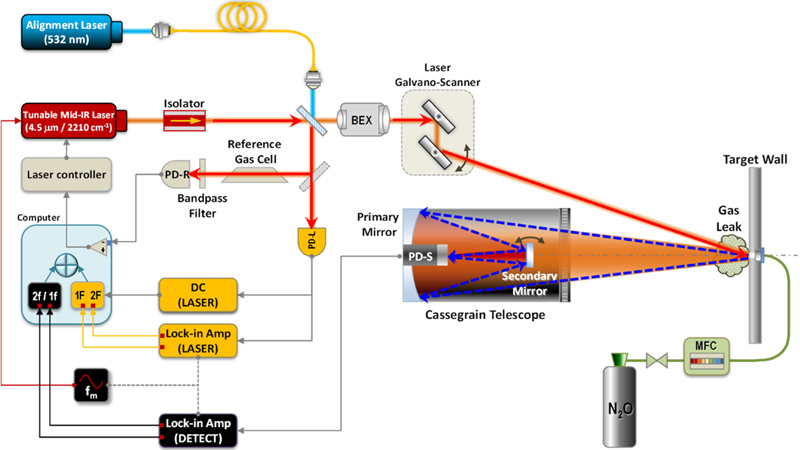
Optical configuration of the gas leak detection system based on frequency modulation absorption spectroscopy (BEX: beam expander, PD-R: photo-diode for reference signal, PD-L: photo-diode for laser source, PD-S: photo-diode for sample signal, MFC: mass flow control, Lock-in Amp: lock-in amplifier)
3.2 아산화질소 누설 가스 검출 테스트 결과
Fig. 6은 아산화질소 가스의 누설 유무에 따른 주파수 변조된 흡수 스펙트럼 파형 변화와 락인앰프로 측정된 변조 주파수별 진폭 변화를 나타내는 결과이다. Fig. 6(a) 왼쪽 파형과 같이 누설 가스가 없는 경우, 기준 아산화질소 가스셀의 공명 흡수선 위치에서 흡수 분광 신호가 전혀 보이지 않고 1f에 해당하는 광량 변조 신호만이 검출됨을 확인할 수 있다. 반면, 누설 가스가 존재하는 경우, 흡수선 위치에서 파형이 변형되어 Fig. 3에서 이론적으로 예측한 형태의 흡수 분광 신호 파형이 관찰됨을 알 수 있다. Fig. 6(b)는 실제로 50 m 거리에 위치한 누설 타깃에서 가스 누설률을 1 sccm으로 설정했을 때 락인앰프를 통해 측정된 1차 및 2차 변조 주파수 성분의 진폭 변화를 나타낸다. 화살표로 표시된 부분이 실제로 누설 가스가 검출된 시점을 나타내며, 1f 신호 크기 대비 2f 신호 크기의 비를 계산함으로써 레이저 광량 변화 영향이 보상된 흡수 분광에 의한 효과만을 확인하고자 하였다. Figs. 7은 5에 나타낸 타깃 상의 누설 위치를 중심으로 빛 조사 위치를 타깃 벽면 상에서 변화시키면서 획득한 영역별 가스 누설 검출 결과를 나타낸다. 각각의 측정 결과는 측정 조건에 해당하는 타깃까지의 거리, 누설률, 스캐닝에 의한 측정 영역(Field of View, FOV)을 변화시켜가면서 획득하였다. 먼저, Fig. 7(a)는 타깃 거리 30 m, 누설률 0.25 sccm, 측정 영역 10 × 10 cm의 조건에서 측정한 결과이다. 그리고, Fig. 7(b)는 타깃 거리 50 m, 누설률 0.01 sccm, 측정 영역 10 × 10 cm의 조건에서 획득되었다. Fig. 7(c)는 타깃 거리 50 m, 누설률 0.005 sccm, 측정 영역 18 × 18 cm에서 획득된 결과이다. 마지막으로 Fig. 7(d)는 타깃 면이 광축에 대하여 10º 기울어진 극한의 경우에서 측정한 결과이다. 이 경우는 측정 조건에 있어서 Fig. 7(c)와 타깃 거리, 누설률은 같았고, 측정 영역만 14 × 14 cm로 조금 줄여 측정하였다. Fig. 7의 측정 결과들은 누설 가스의 농도를 1f 신호에 대한 2f 신호의 크기 비율로 계산하여 상대적인 크기 차이를 쉽게 알아볼 수 있도록 색으로 표시하였다. 주파수 변조로 인한 레이저 자체 광량 변조 성분 크기(Δ1f, Δ2f)는 Fig. 4에 나타낸 바와 같이 락인앰프를 통해 직접적으로 측정이 가능하며, 이에 대한 산란광 측정 신호의 락인앰프 출력 비율을 바로 계산함으로써 누설 가스 흡수선에 의한 2f 변조 신호 영향만을 구별하여 누설 가스의 농도 변화를 판단할 수 있다. 예를 들어, 검은색은 검출된 누설가스가 매우 작은 경우를 나타내며, 붉은색으로 바뀔수록 누설 가스 검출량이 상대적으로 매우 증가하고 있음을 의미한다.
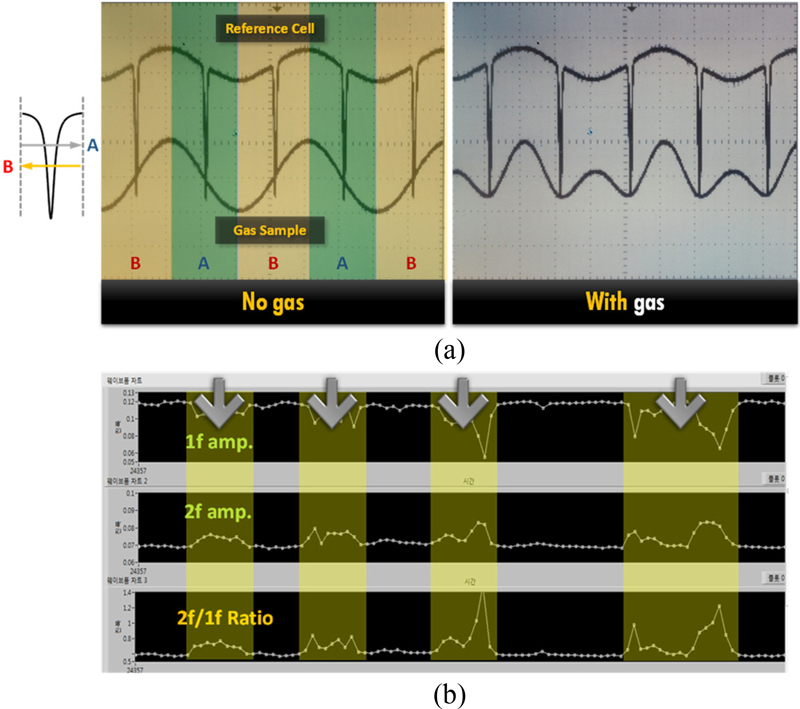
Test results of N2O gas detection (a) frequency modulated absorption spectrum waveform with and without gas leak, (b) flow chart of lock-in amp. in case of 1 sccm gas leak
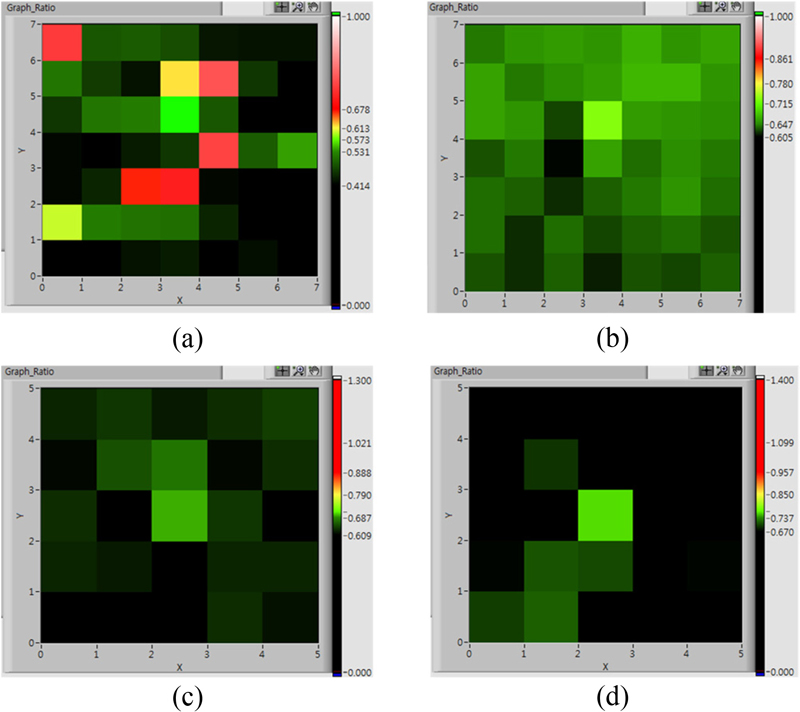
N2O gas leak detection results by area scanning (a) leak rate: 0.25 sccm, target distance: 30 m, FOV(field of view): 10 cm by 10 cm, (b) leak rate: 0.01 sccm, target distance: 50 m, FOV: 10 cm by 10 cm, (c) leak rate: 0.005 sccm, target distance: 50 m, FOV: 18 cm by 18 cm, (d) leak rate: 0.005 sccm, target distance: 50 m, FOV: 14 cm by 14 cm, target inclination angle: 10º
Fig. 7의 결과를 살펴보면, 타깃 거리가 가깝고, 누설률이 높을수록 검출된 신호 크기가 상대적으로 크게 나타나고 있음을 알 수 있다. 또한, 목표로 하는 타깃 거리 50 m에서 0.005 sccm 수준의 극미량의 가스 누설, 타깃 면의 기울기 10º와 같은 극한 조건에서도 Fig. 7(d)와 같이 가스 누설 여부를 판단할 수 있을 정도의 신호 검출이 가능함을 확인할 수 있었다. Table 1은 Fig. 7의 실험 결과를 기반으로 본 연구에서 개발된 주파수 변조 흡수 분광법 기반의 극미량 가스 검출 시스템의 기술 사양을 정리한 것이다.
4. 결론
본 논문에서는 중적외선 레이저의 주파수 변조기반 흡수 분광 측정기술을 이용한 극미량 아산화질소 누설 가스의 원격 검출 방법을 제안하고, 이를 위한 측정 시스템을 구현하였다. 아산화질소 가스 단일 흡수선을 중심으로 4.5 μm QCL 광원의 1 kHz의 주파수 변조 수행을 통해 획득하게 되는 산란광 신호를 분석하여 주파수 변조를 위한 전류 변조에 의해 발생하는 레이저 자체 광량 변화 영향이 보상된 흡수 분광 측정 신호를 획득하였다. 또한, 최대 50 m 떨어진 위치에서의 타깃 면 누설 위치를 중심으로 20 × 20 cm 영역 스캔을 수행하여 0.005 sccm 수준의 극미량 누설 및 타깃 면 기울기 10º와 같은 극한 조건에서도 누설 가스 존재 여부를 판단할 수 있는 고민감도 신호 검출이 가능함을 확인하였다. 본 연구는 수십 m 떨어진 위치에 존재하는 거친 산란면에서 발생하는 극미량 가스 누설의 원격 검출 가능성을 보였으며, 이를 바탕으로 삶의 질 향상을 위한 환경 모니터링 목적으로 다양한 유해가스 검출에도 적용할 수 있을 것으로 기대한다. 또한, 국가 기반 산업인 대형 플랜트 또는 가스 운송용 선박 탱크 내부 누설 위치 원격 검출에도 적용될 수 있을 것으로 기대한다.
Acknowledgments
본 연구는 산업통상자원부 디스플레이 혁신공정 플랫폼 구축 사업 과제(No. 00419221) 지원으로 수행되었습니다.
References
-
Weitkamp, C., (2005), Lidar: range-resolved optical remote sensing of the atmosphere, Springer.
[https://doi.org/10.1007/b106786]
-
Grant, W. B., (1991), Differential absorption and Raman lidar for water vapor profile measurements: a review, Optical Engineering, 30(1), 40-48.
[https://doi.org/10.1117/12.55772]
-
Schotland, R. M., Sassen, K., Stone, R., (1971), Observations by lidar of linear depolarization ratios for hydrometeors, Journal of Applied Meteorology and Climatology, 10(5), 1011-1017.
[https://doi.org/10.1175/1520-0450(1971)010<1011:OBLOLD>2.0.CO;2]
-
Clemitshaw, K., (2004), A review of instrumentation and measurement techniques for ground-based and airborne field studies of gas-phase tropospheric chemistry, Critical Reviews in Environmental Science and Technology, 34(1), 1-108.
[https://doi.org/10.1080/10643380490265117]
-
Dooly, G., Fitzpatrick, C., Lewis, E., (2008), Deep UV based DOAS system for the monitoring of nitric oxide using ratiometric separation techniques, Sensors Actuators B: Chemical, 134(1), 317-323.
[https://doi.org/10.1016/j.snb.2008.05.011]
-
Edner, H., Svanberg, S., Unéus, L., Wendt, W., (1984), Gas-correlation lidar, Optics Letters, 9(11), 493-495.
[https://doi.org/10.1364/OL.9.000493]
-
Iseki, T., Tai, H., Kimura, K., (2000), A portable remote methane sensor using a tunable diode laser, Measurement Science and Technology, 11, 594-602.
[https://doi.org/10.1088/0957-0233/11/6/302]
-
Wojtas, J., Bielecki, Z., Stacewicz, T., Mikolajczyk, J., Medrzycki, R., Rutecka, B., (2011), Application of quantum cascade lasers in nitric oxide and nitrous oxide detection, Acta Physica Polonica A, 120(4), 794-797.
[https://doi.org/10.12693/APhysPolA.120.794]
-
Herriott, D. R., Schulte, H. J., (1965), Folded optical delay lines, Applied Optics, 4(8), 883-889.
[https://doi.org/10.1364/AO.4.000883]
-
Gurlit, W., Zimmermann, R., Giesemann, C., Fernholz, T., Ebert, V., Wolfrum, J., Platt, U., Burrows, J. P., (2005), Lightweight diode laser spectrometer CHILD (compact high-altitude in-situ laser diode) for balloonborne measurements of water vapor and methane, Applied Optics, 44(1), 91-102.
[https://doi.org/10.1364/AO.44.000091]
-
Rothman, L. S., Gordon, I. E., Barbe, A., Benner, D. C., Bernath, P. F., Birk, M., Boudon, V., Brown, L. R., Campargue, A., Champion, J.-P., Chance, K., Coudert, L. H., Dana, V., Devi, V. M., Fally, S., Flaud, J.-M., Gamache, R. R., Goldman, A., Jacquemart, D., Kleiner, I., Lacome, N, Lafferty, W. J., Mandin, J.-Y., Massie, S. T., Mikhailenko, S. N., Miller, C. E., Moazzen-Ahmadi, A., Naumenko, O. V., Nikitin, A. V., Orphal, J., Perevalov, V. I., Perrin, A., Predoi-Cross, A., Rinsland, C. P., Rotger, M., Šimečková, M., Smith, M. A. H., Sung, K., Tashkun, S. A., Tennyson, J., Toth, R. A., Vandaele, A. C., Vander Auwera, J., (2009), The HITRAN 2008 Molecular Spectroscopic Database, Journal of Quantitative Spectroscopy and Radiative Transfer, 110(9-10), 533-572.
[https://doi.org/10.1016/j.jqsrt.2009.02.013]
-
Wang, X.-G., Carrington, T., Tang, J., McKellar, A. R. W., (2005), Theoretical and experimental studies of the infrared rovibrational spectrum of He2-N2O, Journal of Chemical Physics, 123(3), 034301:1-15
[https://doi.org/10.1063/1.1924408]
-
Dutton, C., Sazonov, A., Beaudet, R. A., (1996), High-resolution rovibrational absorption spectrum of CO2-N2O, Journal of Physical Chemistry, 100(45), 17772-17779.
[https://doi.org/10.1021/jp962058z]
-
Bakhirkin, Y. A., Kosterev, A. A., Roller, C., Curl, R. F., Tittel, F. K., (2004), Mid-infrared quantum cascade laser based off-axis integrated cavity output spectroscopy for biogenic nitric oxide detection, Applied Optics, 43(11), 2257-2266.
[https://doi.org/10.1364/AO.43.002257]
-
Sonnenfroh, D. M., Rawlins, W. T., Allen, M. G., Gmachl, C., Capasso, F., Hutchinson, A. L., Sivco, D. L., Baillargeon, J. N., Cho, A. Y., (2001), Application of balanced detection to absorption measurements of trace gases with room-temperature, quasi-CW quantum-cascade lasers, Applied Optics, 40(6), 812-820.
[https://doi.org/10.1364/AO.40.000812]
-
Borri, S., Bartalini, S., de Natale, P., Inguscio, M., Gmachl, C., Capasso, F., Sivco, D. L., Cho, A. Y., (2006), Frequency modulation spectroscopy by means of quantum-cascade lasers, Applied Physics B, 85, 223-229.
[https://doi.org/10.1007/s00340-006-2343-6]
Principal Research Scientist in the Division of Physical Metrology, Korea Research Institute of Standards and Science and a professor in the department of precision measurement at University of Science and Technology. His research interest is optical metrology.
E-mail: jungjae.park@kriss.re.kr
Principal Research Scientist in the Division of Physical Metrology, Korea Research Institute of Standards and Science. His research interest is ultrasensitive laser spectroscopy, photonic quantum computation, nonlinear optical microscopy, super-resolution imaging to length and dimensional metrology.
E-mail: jaeyong@kriss.re.kr
Senior Research Scientist in the Division of Physical Metrology, Korea Research Institute of Standards and Science. Her research interest is dimensional metrology in long-distance and large-scale 3D measurement.
E-mail: jhwoo@kriss.re.kr
Principal Research Scientist in the Division of Physical Metrology, Korea Research Institute of Standards and Science and a professor in the department of precision measurement at University of Science and Technology. His research interest is optical metrology.
E-mail: jonghan@kriss.re.kr