
Experimental Analysis of Performance Variation on Thin Film Solid Oxide Fuel Cell with Different Cathode Area Sizes
Copyright © The Korean Society for Precision Engineering
This is an Open-Access article distributed under the terms of the Creative Commons Attribution Non-Commercial License (http://creativecommons.org/licenses/by-nc/3.0) which permits unrestricted non-commercial use, distribution, and reproduction in any medium, provided the original work is properly cited.
Abstract
To study the geometrical scale dependency of thin film solid oxide fuel cells (SOFCs), we fabricated three thin films SOFCs with the same cross-sectional structure but with different electrode areas of 1, 4 and 9 mm2. Since the activation and ohmic losses of SOFCs depend on their active region, we examined the variations of the power density of the cells with a Pt (anode)/sputtered YSZ/Pt (cathode) structure. We found that a cathode electrode with a low aspect ratio may suffer from high ohmic and activation losses because of the geometrical scale dependency.
Keywords:
Thin film, Solid oxide fuel cell, Scale, Oxygen reduction reaction, Cathode키워드:
박막, 고체산화물연료전지, 크기, 산소환원반응, 공기극1. Introduction
Interest in solid oxide fuel cells (SOFCs), which can operate over 700°C, continues to increase due to their high energy efficiency, flexibility in the choice of fuel, and cost-effective core materials.1-4 Although SOFCs have several excellent qualities, they suffer from slow start-up and shut-down as well as a thermal management problem.5-7 To resolve these problems, several modified SOFCs such as a low-temperature SOFC and a protonic SOFC, have been developed.3,8-10 Thin film SOFCs, which are fabricated using vacuum techniques, are very promising and have been actively researched due to their thin electrolytes, functional layers and electrodes.1,3,11-15
In particular, the ultrathin electrolytes allow the thin film SOFCs to reduce their operating temperature from over 700°C to less than 500°C, which improves the start-up and shut-down function and thermal management of the SOFC system.3,16,17 Various groups proposed free-standing, porous substrate supported, and anode supported thin film SOFCs8,16,18-20 and confirmed their abilities to achieve high power densities and low temperature operation.16 Despite the successful development of the free-standing, porous substrate and anode supported thin film SOFCs for a feasible energy system using low-temperature SOFCs, increasing the size of a membrane electrode assembly (MEA) for stack commercialization is still in its infancy stage due to undesirable effects such as short-circuit of electrolyte and high ohmic polarization of thin electrodes.1,21 As shown in Fig. 1, thin film MEAs with a cm2 area can usually cause high ohmic (In-Plane Direction) resistance within electrodes.10 We need to investigate the geometrical scale dependency of MEAs of different area sizes (see Fig. 1, R1 and R2). In this paper, we designed Pt/YSZ/Pt single cells with different active areas of 1 × 1, 2 × 2, and 3 × 3 mm2. Using experimental methods, we also discovered that the geometrical scale dependency depended on the active area and caused the charge transfer reaction as well as the ohmic resistances.
2. Experimental
The microstructures of the electrolyte and electrode were characterized using a focused ion beam (FIB: Quanta 3D FEG, Operating Voltage: 5 kV), secondary electron microscopy (SEM: Zeiss Sigma, Operating Voltage: 2 kV), X-ray diffraction (XRD, PANalytical X'pert Pro, Netherlands) using Cu Kα radiation and a scanning speed of 3°C min-1 between 20°C and 90°C, and X-ray photoelectron spectroscopy (XPS, Kratos, Japan). As shown in Fig. 2(a), experimental samples of thin film SOFCs were fabricated using a sputtering process. First, under an Ar pressure of 30 mTorr, the porous 300-nm-Pt anode was deposited onto a 100-μm-thick anodic aluminum oxide (AAO) template with 80-nm pores. Then, the 800-nm-YSZ electrolyte was deposited onto a porous Pt anode under a gas mixture of Ar (80%) / O2 (20%) at a pressure of 5 mTorr. Finally, the sporous 200-nm-thick Pt cathode was also deposited using sputtering under an Ar pressure of 90 mTorr. Current-voltage-power (I-V-P) curves and electrochemical impedance spectroscopy (EIS) values were measured using a Solartron 1287 / 1260 module. The voltage sweep rates for the potentiodynamic and the perturbation voltages of the EIS were 10 mVs-1 and 30 mV, respectively. The frequency range for the E IS measurements is 105-10Hz. Fig. 2(a) shows a schematic of the system operating on H2 (20 sccm) at the anode and the air-breathing system at the cathode. The thermocouple was placed on the air side of the Pt cathode.
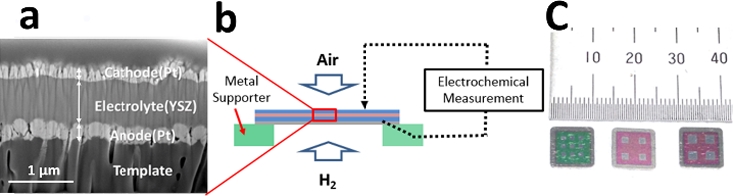
(a) Cross-sectional SEM image of thin film SOFC, (b) Experimental set-up of thin film SOFC through electrochemical measurement, (c) Image of samples used in experiments of cathode areas of 1, 4 and 9 mm2
The heating rate of the customized test station was 6°C/min and the final target temperature for the measurement was 500°C; the details on the test station’s setup and sequence have been previously reported.9,17 Besides, a sealant (CP 4010, Aremco Products Inc.) was used to ensure the gas tightness between the metal supporter and the sample. Fig. 2(a) shows the single fabricated cells with three different active areas of 1, 4, and 9 mm2. Figs. 3(a) and 3(b) show the yttria-stabilized zirconia (YSZ) results by X-ray diffraction and XPS, respectively. Fig. 3(a) shows that the sputtered YSZ has clear XRD patterns of (111), (200), (220), and (311). As shown in Fig. 3(a), we confirmed a mole doping rate of 8 from the deposited YSZ thin film through XPS.
3. Results and Discussion
Fig. 4(a) shows the I-V-P curves of three cells with active areas of 1, 4 and 9 mm2. Even though structures of the three cells are the same except for the active cathode areas, the maximum power densities (MPDs) of the three cells with active areas of 1, 4, and 9mm2 and the cathode active areas are significantly different at 500°C, (137, 68, 39 mW/cm2, respectively). We assume the intrinsic defects of manufactured cells are negligible from the sputtering process. Furthermore, this phenomenon completely attributed to the in-plane resistance variations at the cathode, which are due to the Faradaic impedance and ohmic polarization change. As shown in Fig. 4(a), the EIS shows that both the ohmic resistance (X-Intercept) and Faradaic resistance (Semi-Circle) decrease as the active area decreases from 9 mm2 to 1 mm2. It is widely known that Faradaic resistance arises from oxygen reduction reactions (ORRs).22
The non-uniform distribution of electrons within the thin cathode (< 200 nm) makes it hard for reaching the YSZ/Pt electrochemical interface for ORR from the micro-probe, resulting in the variations of ohmic polarization: 0.91 Ωcm2 (1mm2), 2.21 Ωcm2 (4 mm2), and 2.89 Ωcm2 (9 mm2) in Fig. 3(a). As stated above, we believe that the ohmic loss is due to the localized non-uniform electron distribution within the cathode electrode. Second, it is generally known that the low-frequency arcs in EIS typically indicate a sluggish ORR at the cathode.23 Among the ORR steps, Eqs. (1) and (2), in which electrons participate significantly, influence oxygen incorporation at the cathode in the following way.24
(1) |
(2) |
As a characteristic of thin film SOFCs with very thin electrodes, a triple phase boundary (TPB) is localized due to the localized electron distribution at the cathode, and the activation polarization (i.e. ORR at the Cathode) increases, as shown in Eqs. (1) and (2). Park et al. observed that the MPDs differed according to the patterns of current collectors at the cathode only, although the cell architectures were the same.17 Thus, we assume that the in-plane resistance strongly occurs within a thin film SOFC with wider cathode (Fig. 1). Many studies reported that the MPDs of thin film SOFCs differ due to the geometrical scale dependency of different active areas, despite having similar platforms and structures.11,13,14,22 Fig. 5 exhibits the power density variations to the aspect ratio, which is the following relation,
(3) |
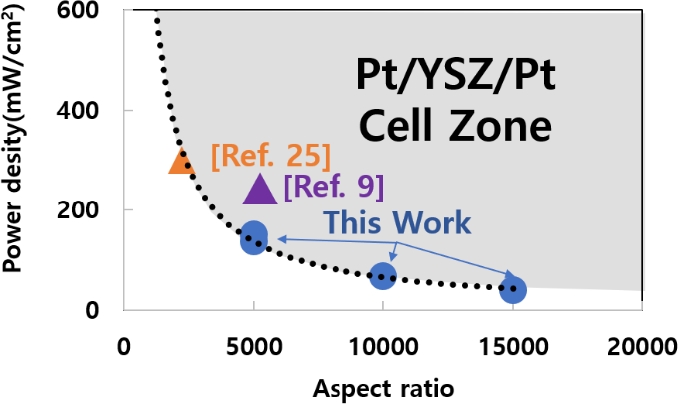
Power density variation with respect to aspect ratio and its inset graph. 5000 (1 mm2), 10000 (4 mm2), and 15000 (9 mm2)
Where the cathode is 200-nm-thick, and the areas are 1, 4, and 9mm2. As shown in Fig. 5, the power densities dramatically decrease up to the aspect ratio of 5000, and then the power densities are stabilized over the aspect ratio of 10000. Chang et al. reported that the aspect ratio of the area of 1 mm2 to the cathode of a 200-nm-thick Pt is also 5000.9 However, Tsuchiya et al. reported that the aspect ratio of area of the 25 mm2 to the cathode with a 47-nm-thick LSCF and a 1.5-μm-thick Pt grid is 3232.25 Although their group developed a new architecture with the Pt grid cathode for scaling up active area of the thin film SOFC through micro-/nano-fabrications, it seems that the optimization of thin film SOFC is not enough in Fig. 5.25 In the same time, the power density of 300 mW/cm2 was measured at 750°C. Fig. 5 shows the thin film SOFC from Lee et al. has better architecture at the cathode than that of Tsuchiya et al.25,26 Also, we draw the reference trendline from experimental results and literatures.25,26 It is believed that the gray zone is the well-optimized thin film SOFC with Pt / YSZ / Pt.
4. Conclusion
We studied the geometrical scale dependency of the thin film SOFCs using experimental approaches. Although the cross-sectional structure of the single cells was the same, the overall MPDs of cells with active areas of 1, 4, and 9 mm2 were different due to the geometrical scale dependency. Optimizing the current collectors and the cathode structures (i.e., Porosity and Thickness) is strongly recommended for increasing the size of thin film SOFCs and reducing the ORR and ohmic polarization.
Acknowledgments
This paper was supported by Wonkwang University in 2019.
REFERENCES
-
Chang, I., Bae, J., Park, J., Lee, S., Ban, M., et al., “A Thermally Self-Sustaining Solid Oxide Fuel Cell System at Ultra-Low operating Temperature (319°C) Energy,” Vol. 104, pp. 107-113, 2016.
[https://doi.org/10.1016/j.energy.2016.03.099]
-
Hibino, T., Hashimoto, A., Inoue, T., Tokuno, J. I., Yoshida, S. I., et al., “A Low-Operating-Temperature Solid Oxide Fuel Cell in Hydrocarbon-Air Mixtures,” Science, Vol. 288, No. 5473, pp. 2031-2033, 2000.
[https://doi.org/10.1126/science.288.5473.2031]
-
Park, J., Lee, Y., Chang, I., Cho, G. Y., Ji, S., et al., “Atomic Layer Deposition of Yttria-Stabilized Zirconia Thin Films for Enhanced Reactivity and Stability of Solid Oxide Fuel Cells," Energy, Vol. 116, pp. 170-176, 2016.
[https://doi.org/10.1016/j.energy.2016.09.094]
-
Steil, M., Nobrega, S., Georges, S., Gelin, P., Uhlenbruck, S., et al., “Durable Direct Ethanol Anode-Supported Solid Oxide Fuel Cell,” Applied Energy, Vol. 199, pp. 180-186, 2017.
[https://doi.org/10.1016/j.apenergy.2017.04.086]
-
Mounir, H., Belaiche, M., El Marjani, A., and El Gharad, A., “Thermal Stress and Probability of Survival Investigation in a Multi-Bundle Integrated-Planar Solid Oxide Fuel Cells IP-SOFC (Integrated-Planar Solid Oxide Fuel Cell),” Energy, Vol. 66, pp. 378-386, 2014.
[https://doi.org/10.1016/j.energy.2014.01.017]
-
Safa, Y., Hocker, T., Prestat, M., and Evans, A., “Post-Buckling Design of Thin-Film Electrolytes in Micro-Solid Oxide Fuel Cells,” Journal of Power Sources, Vol. 250, pp. 332-342, 2014.
[https://doi.org/10.1016/j.jpowsour.2013.10.125]
-
Evans, A., Bieberle-Hütter, A., Galinski, H., Rupp, J. L., Ryll, T., et al., “Micro-Solid Oxide Fuel Cells: Status, Challenges, and Chances,” Monatshefte für Chemie-Chemical Monthly, Vol. 140, No. 9, pp. 975-983, 2009.
[https://doi.org/10.1007/s00706-009-0107-9]
-
Chang, I., Heo, P., and Cha, S. W., “Thin Film Solid Oxide Fuel Cell Using a Pinhole-Free and Dense Y-Doped BaZrO3,” Thin Solid Films, Vol. 534, pp. 286-290, 2013.
[https://doi.org/10.1016/j.tsf.2013.03.024]
-
Chang, I., Ji, S., Park, J., Lee, M. H., and Cha, S. W., “Ultrathin YSZ Coating on Pt Cathode for High Thermal Stability and Enhanced Oxygen Reduction Reaction Activity,” Advanced Energy Materials, Vol. 5, No. 10, Paper No. 1402251, 2015.
[https://doi.org/10.1002/aenm.201402251]
-
Park, J., Lee, Y., Chang, I., Lee, W., and Cha, S. W., “Engineering of the Electrode Structure of Thin Film Solid Oxide Fuel Cells,” Thin Solid Films, Vol. 584, pp. 125-129, 2015.
[https://doi.org/10.1016/j.tsf.2014.11.018]
-
Patil, T. C. and Duttagupta, S. P., “Micro-Solid Oxide Fuel Cell: A Multi-Fuel Approach for Portable Applications,” Applied Energy, Vol. 168, pp. 534-543, 2016.
[https://doi.org/10.1016/j.apenergy.2016.01.111]
-
Li, Y., Wong, L. M., Yu, C. C., Wang, S., and Su, P. C., “Pulsed Laser Deposition of Ba0.5Sr0.5Co0.8Fe0.2O3−δ Thin Film Cathodes for Low Temperature Solid Oxide Fuel Cells,” Surface and Coatings Technology, Vol. 320, pp. 344-348, 2017.
[https://doi.org/10.1016/j.surfcoat.2016.12.051]
-
Vaßen, R., Hathiramani, D., Mertens, J., Haanappel, V., and Vinke, I., “Manufacturing of High Performance Solid Oxide Fuel Cells (SOFCs) with Atmospheric Plasma Spraying (APS),” Surface and Coatings Technology, Vol. 202, No. 3, pp. 499-508, 2007.
[https://doi.org/10.1016/j.surfcoat.2007.06.064]
-
Marchand, O., Bertrand, P., Mougin, J., Comminges, C., Planche, M. P., et al., “Characterization of Suspension Plasma-Sprayed Solid Oxide Fuel Cell Electrodes,” Surface and Coatings Technology, Vol. 205, No. 4, pp. 993-998, 2010.
[https://doi.org/10.1016/j.surfcoat.2010.06.001]
-
Bae, J., Lee, D., Hong, S., Yang, H., and Kim, Y. B., “Three-Dimensional Hexagonal GDC Interlayer for Area Enhancement of Low-Temperature Solid Oxide Fuel Cells,” Surface and Coatings Technology, Vol. 279, pp. 54-59, 2015.
[https://doi.org/10.1016/j.surfcoat.2015.07.066]
-
An, J., Kim, Y. B., Park, J., Gür, T. M., and Prinz, F. B., “Three-Dimensional Nanostructured Bilayer Solid Oxide Fuel Cell with 1.3 W/cm2 at 450°C,” Nano Letters, Vol. 13, No. 9, pp. 4551-4555, 2013.
[https://doi.org/10.1021/nl402661p]
-
Park, J., Chang, I., Paek, J. Y., Ji, S., Lee, W., et al., “Fabrication of the Large Area Thin-Film Solid Oxide Fuel Cells,” CIRP Annals, Vol. 63, No. 1, pp. 513-516, 2014.
[https://doi.org/10.1016/j.cirp.2014.03.065]
-
Muecke, U. P., Beckel, D., Bernard, A., Bieberle-Hütter, A., Graf, S., et al., “Micro Solid Oxide Fuel Cells on Glass Ceramic Substrates,” Advanced Functional Materials, Vol. 18, No. 20, pp. 3158-3168, 2008.
[https://doi.org/10.1002/adfm.200700505]
-
Park, J., Paek, J. Y., Chang, I., Ji, S., Cha, S. W., et al., “Pulsed Laser Deposition of Y-doped BaZrO3 Thin Film as Electrolyte for Low Temperature Solid Oxide Fuel Cells,” CIRP Annals, Vol. 62, No. 1, pp. 563-566, 2013.
[https://doi.org/10.1016/j.cirp.2013.03.025]
-
Joo, J. H. and Choi, G. M., “Simple Fabrication of Micro-Solid Oxide Fuel Cell Supported on Metal Substrate,” Journal of Power Sources, Vol. 182, No. 2, pp. 589-593, 2008.
[https://doi.org/10.1016/j.jpowsour.2008.03.089]
-
Lawlor, V., Griesser, S., Buchinger, G., Olabi, A., Cordiner, S., et al., “Review of the Micro-Tubular Solid Oxide Fuel Cell: Part I. Stack Design Issues and Research Activities,” Journal of Power Sources, Vol. 193, No. 2, pp. 387-399, 2009.
[https://doi.org/10.1016/j.jpowsour.2009.02.085]
-
O’hayre, R., Cha, S. W., Colella, W., and Prinz, F. B., “Fuel Cell Fundamentals,” John Wiley & Sons, 2nd Ed., 2016.
[https://doi.org/10.1002/9781119191766]
-
Adler, S. B., “Factors Governing Oxygen Reduction in Solid Oxide Fuel Cell Cathodes,” Chemical Reviews, Vol. 104, No. 10, pp. 4791-4844, 2004.
[https://doi.org/10.1021/cr020724o]
-
Shim, J. H., Park, J. S., Holme, T. P., Crabb, K., Lee, W., et al., “Enhanced Oxygen Exchange and Incorporation at Surface Grain Boundaries on an Oxide Ion Conductor,” Acta Materialia, Vol. 60, No. 1, pp. 1-7, 2012.
[https://doi.org/10.1016/j.actamat.2011.09.050]
-
Tsuchiya, M., Lai, B. K., and Ramanathan, S., “Scalable Nanostructured Membranes for Solid-Oxide Fuel Cells,” Nature Nanotechnology, Vol. 6, No. 5, pp. 282-286, 2011.
[https://doi.org/10.1038/nnano.2011.43]
-
Noh, H. S., Yoon, K. J., Kim, B. K., Je, H. J., Lee, H. W., et al., “Thermo-Mechanical Stability of Multi-Scale-Architectured Thin-Film-based Solid Oxide Fuel Cells assessed by Thermal Cycling Tests,” Journal of Power Sources, Vol. 249, pp. 125-130, 2014.
[https://doi.org/10.1016/j.jpowsour.2013.10.101]
Assistant Professor in the Department of Automotive Engineering, Yeungnam University. His research interests includes low temperature SOFCs, thin film mechanics and fundamental property characterizations.
E-mail: jdbaek@yu.ac.kr
Assistant Professor in the Department of Automotive Engineering, Wonkwang University. His research interest is fuel cell, thin film, and battery.
E-mail: ikwhang2@wku.ac.kr