
고령자의 COM-COP 경사각 변화에 따른 보행 안정성 비교 연구
Copyright © The Korean Society for Precision Engineering
This is an Open-Access article distributed under the terms of the Creative Commons Attribution Non-Commercial License (http://creativecommons.org/licenses/by-nc/3.0) which permits unrestricted non-commercial use, distribution, and reproduction in any medium, provided the original work is properly cited.
Abstract
The current method of gait analysis has several limitations for determining gait stability, such as a complicated preparation process, repeated experimental procedures that are time-consuming, and financial burden of experiments. This study investigated whether gait stability could be analyzed using only the COM-COP (Center of Mass-Center of Pressure) inclination angle connecting COM and COP. COM and COP coordinates were obtained from a motion analysis system for a total of 40 elderly and young subjects. The COM-COP inclination angle that changed in real time during level walking was then analyzed to obtain gait stability on each of sagittal and frontal planes using these coordinates. As a result, the gait symmetry index on the sagittal plane did not show a statistically significant difference between young and elderly subjects (First Step, p = 0.189; Second Step, p = 0.711). On the frontal plane, elderly subjects showed 0.39 degrees (p = 0.058) and 0.5 degree (p = 0.03) larger side-to-side sway angles in the first and second steps than young subjects, respectively. Gait stability can be analyzed using a more simplified experimental method with minimum amount of data in future gait analysis.
Keywords:
COM-COP inclination angle, Gait symmetry index, Gait stability, Elderly키워드:
COM-COP 경사각, 보행 대칭 지수, 보행 안정성, 고령자1. 서론
보행은 인간이 살아가면서 가장 기본적인 이동 수단이며 일상생활을 수행하는데 있어 매우 중요한 역할을 한다. 인간은 오랜 기간 훈련에 걸쳐 인체의 자유도를 활용하여 다양한 환경에서 건강하고 안정적인 보행을 습득하며, 이는 일상생활을 더욱 쾌적하게 한다. 이러한 보행 안정성을 분석하기 위한 방법으로는 크게 시각적 관찰을 통한 것과 생역학적 측정을 이용하는 두 가지 방법이 있는데,1-3 최근에는 보행 안정성 분석 시 생역학적 측정을 위해 3차원 동작분석시스템을 활용한 연구들이 활발하게 진행되고 있다.
기존 보행 안정성 연구에서는 개인 마다의 연령, 신장 및 체중 등의 인적 사항을 기반으로 보폭(Stride Length), 보행속도(Gait Velocity), 양발너비(Stride Width), 보행률(Cadence), 발 각도(Foot Angle) 등의 변수가 복합적으로 적용된다.4-8 예를 들어, 고령자들의 경우 노화로 인해 균형 능력이 감소하거나 근력의 약화와 같은 신체적인 기능 상실이 발생함으로써 낙상 위험의 증가와 보행의 안정성이 감소한다.4 때문에 고령자들은 보행 시 안정성을 증가시키기 위해 젊은 성인들과 비교하여 상대적으로 보폭과 보행속도가 감소하고, 두 발의 지지 시간(Double-Support Time)이 증가한다고 보고된 바 있으며,4,5 BMI가 증가할수록 발 각도가 증가하며, 반대로 보폭과 보행속도는 감소하였다고 알려졌다.6-8 이 밖에 다른 선행연구에서는 보행 안정성을 평가하기 위해 평지 보행 중 발 궤적(Foot Trajectory)의 가변성과 동적 안정성에 대한 Cohen’s d 분석 등을 진행하였다.35-38 이처럼 기존 연구에서는 보행 안정성을 분석하기 위해 다양한 변수들의 지표를 가지고 연구들이 진행되었다.
보행 안정성 분석을 위해 제한된 보행 환경, 보행 시 관절 각도의 범위, 근전도(Electromyography, EMG) 신호를 통한 보행 안정성 분석 등 다양한 운동학적(Kinematic) 데이터를 이용한 연구가 활발하게 이루어지고 있다. Ko 등은 동작분석시스템을 이용하여 보행 시 보행속도에 따라 하퇴부의 생체역학적 분석을 통해 무릎 골관절염(Knee-Osteoarthritis)과 관련된 특정 보행 패턴을 식별하는 연구를 수행하였으며,9 Abbass 등은 디지털 비디오 카메라 레코더를 사용하여 보행 주기별로 슬관절 및 고관절의 각도를 통해 보행 안정성을 분석하였다.10 다른 선행연구에서는 보행 시 측정된 EMG 신호의 근활성도를 통해 근육 활동을 비교하고, 보행 안정성을 분석하고자 하였다.1,11,12
보행 시 실시간으로 변화하는 무게 중심(Center of Mass, COM)과 압력중심(Center of Pressure, COP)은 보행 안정성을 분석하는데 중요한 요소 중 하나이다.13-15 보행 분석 시 기존 연구들의 단점을 극복하기 위한 개선연구로 COM과 COP를 이용한 보행 안정성을 분석한 연구들이 소개되었다. Yamaguchi 등은 시상면에서의 COM-COP 접선 각도와 초기 접촉(Initial Contact) 구간의 마찰계수를 통해 보행속도와 보폭 길이를 분석하였으며,16 Meurisse 등은 보행속도와 연령별로 COM의 움직임 궤적을 통해 보행 단계를 예측하고자 하였다.17 또한 Krebs 등은 한발 지지(Single Support) 시 COM과 COP 사이의 최대 수평거리를 통해 보행 안정성을 평가하였다.18 하지만, 단순히 COM과 COP 사이의 수평거리만을 통해 보행 안정성을 평가하기에는 보행자의 신장에 따른 COM과 COP 사이의 수직거리에 대한 영향을 배제하였다. 이를 개선하기 위한 연구로 COM과 COP를 연결하여 COM-COP 경사각도를 고려한 보행 안정성을 평가하였다.19-23 하지만, 이러한 기존 연구들도 보행 안정성을 분석하기 위해서 특정 변수들을 지표화하지 못한 채 복잡한 분석 과정을 통한 연구가 진행되고 있다.
본 연구에서는 보다 간소화된 분석 과정을 통해 평지 보행 시 실시간으로 변화하는 COM과 COP의 경사각도를 통해 연령별로 보행 안정성 판별 가능성을 확인하고자 하였다. 그리고 시상면과 관상면에서 나타난 COM과 COP의 경사각도를 통해 보행 안정성을 비교분석하고자 하였다.
2. 연구 대상 및 방법
2.1 피험자 및 실험 과정
근골격계 질환이 없는 젊은 성인 19명(Age: 25.95±1.29, Height: 170.61±3.68, Weight: 66.18±5.41)과 고령자 21명(Age: 71.43±2.19, Height: 161.31±3.95, Weight: 61.43±4.03)을 대상으로 자연스러운 보행으로 3회씩 보행 실험을 실시하였다. 젊은 성인과 고령자를 다시 체질량지수(Body Mass Index, BMI)로 나누었으며, BMI 범위는 정상체중(Normal, 18.5 kg/m2 < BMI < 22.9 kg/m2), 과체중(Overweight, 23 kg/m2< BMI < 24.9 kg/m2), 비만체중(Obese Weight, 25 kg/m2< BMI)까지 세 가지로 나누었다(Table 1).
보행 분석을 위한 측정도구는 2개의 힘 측정판(Kistler, Instrumemt Corp. & AMTI Inc., USA), 적외선 카메라 8대(Oespery, Motion Analysis, USA)와 10 mm의 반사 마커를 사용하였으며, 카메라 속도는 60 frames/sec로 설정하였다. 피험자는 Helen Hayes Marker Set을 이용하여 총 36개의 반사 마커(좌우 Toe 2개, 좌우 Heel 2개, 좌우 Ankle 2개, 좌우 Ankle Medial 2개, 좌우 Shank 2개, 좌우 Knee 2개, 좌우 Knee Medial 2개, 좌우 Thigh 2개, Anterior and Post Superior Iliac Spine 4개, 좌우 Hand 2개, 좌우 Wrist 2개, 좌우 Wrist Medial 2개, 좌우 Elbow 2개, 좌우 Elbow Medial 2개, 좌우 Shoulder 2개, Offset 1개, Rear, Top, Front Head 3개)를 인체에 부착하였으며,39 외부환경에 의해 생길 수 있는 오차를 최소화하기 위해 교정(Calibration)을 진행하였다.23 동작분석프로그램(Cortex, Motion analysis, USA)을 통해 평지 보행 시 실시간으로 변화되는 COM, 힘 측정판에서 측정되는 COP의 위치를 좌표화하여 기록하였다.
2.2 COM-COP 경사각도 계산 방법
동작분석시스템을 통해 확보된 COM 좌표(XCOM, YCOM, ZCOM)와 COP 좌표(XCOP, YCOP, ZCOP (= 0))를 다음의 공식을 통해 COM-COP 경사각도를 계산하였다(Fig. 1).21 COM-COP 경사 각도는 COM의 좌표 위치에서 COP의 좌표 위치 사이를 이은 선과 COM의 좌표 위치에서 수직으로 내린 두 선 사이의 각도를 나타내며, 각 시상면과 관상면에서 COM-COP 경사각도를 표현하였다(Fig. 2).
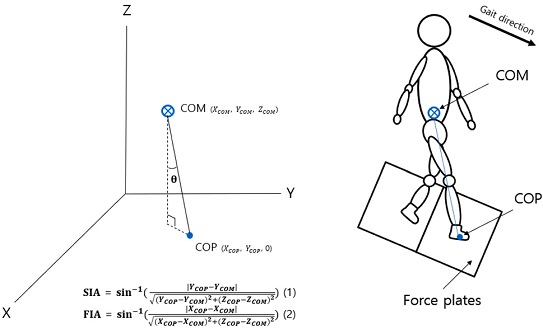
Calculation process of COM-COP inclination angle (a) COM-COP inclination angle formula of sagittal plane, and (b) COM-COP inclination angle formula in frontal plane
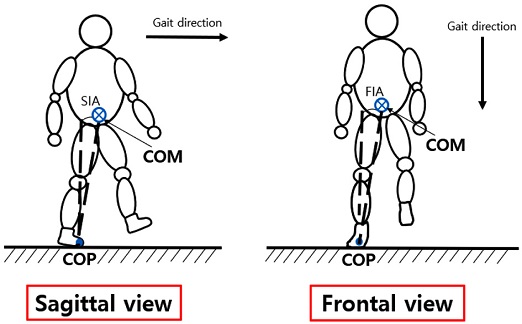
Schematization showing the COM-COP inclination angle (a) Left: Sagittal view, and (b) Right: Frontal view
3차원 COM-COP 경사각도에서 시상면에서 보았을 때의 COM-COP 경사각도를 Sagittal Inclination Angle (SIA)라고 정의하였으며, 관상면에서 보았을 때의 COM-COP 경사각도를 Frontal Inclination Angle (FIA)라고 정의하였다. 시상면에서 보았을 때 COM과 COP의 경사각도가 0o인 중간지지기(Midstance) 지점을 기준으로 수선의 발을 그어 다시 두 가지로 경사각도를 나누어 정의하였는데, 발뒤축접지(Heel Strike) 구간에서 Midstance 구간까지의 각도를 전방 경사각도(Anterior Sagittal Inclination Angle, ASIA), Midstance 구간에서 발가락 떼기(Toe Off) 구간까지를 후방 경사각도(Posterior Sagittal Inclination Angle, PSIA)로 정의하였다(Fig. 3). 관상면에서 보았을 때 Heel Strike 구간에서 Toe Off 구간까지 좌우로 벌어지는 최대 경사각도를 Maximum Frontal Inclination Angle (Max FIA), 최소 경사각도를 Minimum Frontal Inclination Angle (Min FIA)로 정의하였다(Fig. 3).
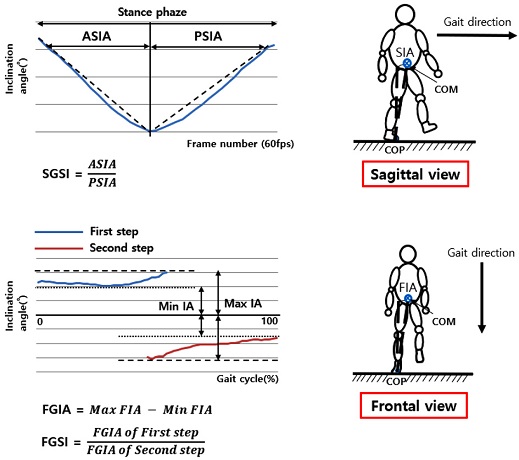
Definition of anterior sagittal inclination angle (ASIA), posterior sagittal inclination angle (PSIA) and sagittal gait symmetry index (SGSI); The angle from the heel strike phase to the midstance phase was defined as the ASIA and the angle from the midstance phase to the toe-off phase was defined as the PSIA (Top view). Definition of maximum frontal inclination angle (Max FIA), minimum frontal inclination angle (Min FIA), frontal gap inclination angle (FGIA) and frontal gap symmetry index (FGSI)
2.3 COM-COP 경사각을 고려한 보행 분석
보행 시 피험자의 우세다리(Dominant Leg)를 기준으로 우세보(First Step), 비우세보(Second Step)으로 나누었다. 시상면과 관상면에서 각각의 COM-COP 경사각도를 계산한 뒤, 시상면에서는 Midstance를 기준으로 정의된 ASIA와 PSIA를 활용하였다. ASIA를 PSIA로 나누어 First Step과 Second Step의 시상면 보행 대칭 지수(Sagittal Gait Symmetry Index, SGSI)를 계산하였으며, 이를 통해 시상면에서 First Step과 Second Step의 보행 안정성을 비교분석 하였다(Fig. 3). 관상면에서는 보행 시 COM과 COP 사이의 좌우로 벌어지는 Max FIA와 Min FIA를 활용하였다. Max FIA와 Min FIA의 차이 값(Frontal Gap Inclination Angle, FGIA)을 계산하였으며, First Step과 Second Step의 FGIA를 비교하여 First Step의 FGIA에서 Second Step의 FGIA를 나누어 관상면 보행 대칭 지수(Frontal Gait Symmetry Index, FGSI)를 계산하였으며, 이를 통해 관상면에서의 보행 안정성을 비교분석 하였다(Fig. 3). 이를 젊은 성인, 고령자 두 가지의 연령별로 3가지의 BMI로 나누어 보행 안정성을 분석하고자 하였다. 본 연구에서는 같은 연령에서 3가지 BMI별간 유의한 차이를 확인하기 위하여 각각 One-Way ANOVA를 시행하였고, 같은 BMI에서 두 가지 연령별로 유의한 차이를 확인하기 위해 각각 T-Test(독립표본 T검정)를 실시하였으며, 이때 통계적 유의수준은 0.05로 하였으며, Type I Error를 통제하기 위해 Bonferroni Correction 분석법을 적용하였다. 그리고 FGSI와 BMI와의 관계를 확인하기 위해 Microsoft Excel에서 제공하는 회귀분석과 상관분석을 적용하였다.
3. 연구 결과
3.1 시상면의 COM-COP 경사각도
젊은 성인과 고령자의 BMI별 First Step과 Second Step에서 ASIA와 PSIA을 비교하였을 때, 가장 큰 각도는 46.83o로 나타났으며, 가장 작은 각도는 39.18o로 나타났다. First Step의 ASIA에서 연령별로 비교할 때, 3가지 모든 BMI군에서 고령자가 젊은 성인보다 크게 나타났으며 평균적으로 유의한 차이(p < 0.001)를 보였다. 또한 젊은 성인의 First Step의 ASIA에서 BMI별로 유의한 차이(p = 0.022)를 보였다. 평균적으로 고령자가 젊은 성인의 First Step PSIA보다 1.84o만큼 크게 나타났으며, 이때 유의한 차이(p = 0.023)를 보였다. 또한 고령자의 Second Step의 ASIA에서 BMI별로 유의한 차이(p = 0.021)를 보였고, 평균적으로 고령자의 Second Step의 ASIA, PSIA이 젊은 성인의 보다 각각 2.39, 2.64o만큼 크게 나타났으며, 유의한 차이(각각 p = 0.001, p < 0.001)를 보였다. 결과적으로 BMI별 First Step과 Second Step의 모든 경우에서 고령자가 젊은 성인보다 크게 나타났으며, 유의한 차이를 보였다. 그리고 시상면에서 ASIA, PSIA를 비교할 때, 평균적으로 ASIA가 PSIA보다 높게 나타났다(Fig. 4).
3.2 관상면의 COM-COP 경사각도
젊은 성인과 고령자의 BMI별 First Step과 Second Step에서 Max FIA와 Min FIA을 비교하였을 때, 가장 큰 각도는 7.22o로 나타났으며, 가장 작은 각도는 2.07o로 나타났다. 평균적으로 First Step의 Max FIA에서 고령자가 젊은 성인보다 0.87o만큼 크게 나타났으며, 유의한 차이(p = 0.002)를 보였다. First Step의 Min FIA에서 연령별로 비교할 때 평균적으로 고령자가 젊은 성인보다 0.48o만큼 크게 나타났으며, 유의한 차이(p = 0.011)를 보였다.
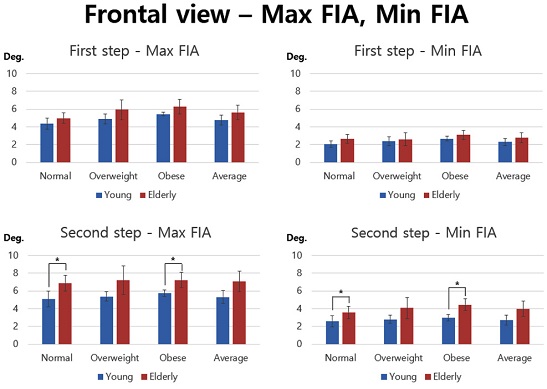
Comparison of maximum frontal inclination angle (Max FIA) and minimum frontal inclination angle (Min FIA) of the young subjects and the elderly subjects between the first step and the second step by each body mass index (*: p < 0.05) in the frontal view
Second Step의 Max FIA에서 연령별로 비교할 때, 세 가지 모든 BMI군에서 고령자가 젊은 성인보다 크게 나타났으며, 평균적으로 유의한 차이(p < 0.0001)를 보였다. Second Step의 Min FIA에서 연령별로 비교할 때, 과체중을 제외한 나머지 BMI군에서 고령자가 젊은 성인보다 크게 나타났고, 유의한 차이(정상체중, 비만체중, 평균 각각 p = 0.011, p = 0.002, p < 0.0001)를 보였다.
3.3 시상면과 관상면에서의 보행 대칭지수
시상면에서 젊은 성인과 고령자의 BMI별 SGSI (= ASIA/PSIA)를 비교한 결과 First Step과 Second Step의 SGSI은 모든 경우에서 젊은 성인과 고령자간 통계적으로 유의한 차이는 보이지 않았다(First Step p = 0.189, Second Step p = 0.711) (Fig. 6).
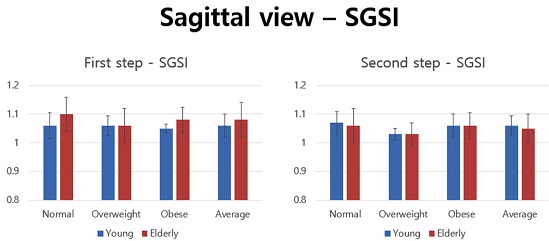
Comparison of sagittal gait symmetry index (SGSI) of the young subjects and the elderly subjects between the first step and the second step by each body mass index (*: p < 0.05) in the sagittal view
관상면에서 젊은 성인과 고령자의 BMI별 FGIA (Max FIA-Min FIA)를 비교하였을 때, First Step과 Second Step에서 고령자가 젊은 성인보다 평균 FGIA이 크게 나타났으며, 그 중 Second Step에서 유의한 차이(p = 0.03)가 나타났다. 그리고 First Step의 FGIA에서 고령자의 경우 젊은 성인과 비교하여 평균적으로는 크게 나타났으나, 유의한 차이는 나타나지 않았다(p = 0.058). 그리고 Second Step의 FGIA에서 고령자의 경우 젊은 성인과 비교하였을 때 평균적으로 크게 나타났으며, 유의한 차이가 나타났다(p = 0.03). FGSI를 비교하였을 때, 고령자의 경우 BMI별로 유의한 차이(p = 0.023)가 나타났다(Fig. 7). 젊은 성인과 고령자의 BMI별 FGSI (FGIA of First Step/FGIA of Second Step)를 비교하였을 때, 통계적으로 유의한 차이는 없었다(p = 0.677). 또한 BMI가 증가할수록 고령자의 FGSI가 커졌으며, BMI가 변화할수록 FGSI가 젊은 성인보다 고령자층에서 민감하게 변화하였다. 이는 결과적으로 BMI와 FGSI의 관계에서 젊은 성인은 y = 0.0231x + 0.5897(R = 0.134, p = 0.705), 고령자는 y = 0.1257x - 1.7993(R = 0.513, p = 0.155)로 고령자 그룹에서 약한 상관관계를 보였다(Fig. 8).
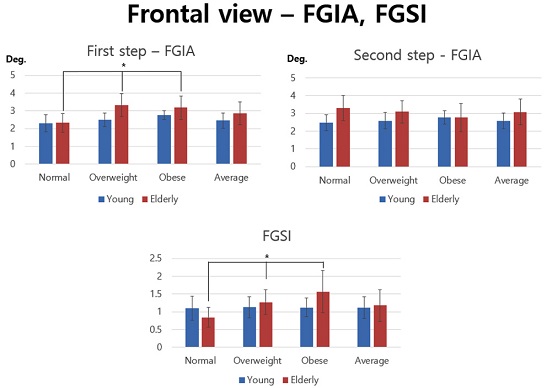
Comparison of frontal gap inclination angle (FGIA) and frontal gap symmetry index (FGSI, FGIA of first step/FGIA of second step) of the young subjects and the elderly subjects between the first step and the second step by each body mass index (*: p < 0.05) in the frontal view
4. 토의
본 연구에서는 동작분석시스템을 통해 확보한 COM 좌표와 COP 좌표를 통해 평지 보행 시 실시간으로 변화하는 COM-COP 경사각도를 계산하였고, 계산된 COM-COP 경사각도를 통해 젊은 성인과 고령자 두 연령대의 시상면과 관상면에서의 보행 대칭 지수를 계산하여 서로 비교하였다.
본 연구에서는 보다 간소화된 분석 과정을 보행 안정성 분석에 도입하기 위하여 COM-COP 경사각도의 새로운 지표를 통해 보행 안정성의 판별이 가능한지를 확인하고자 하였으며, 젊은 성인과 고령자를 대상으로 한 보행 분석을 통해 이를 검증해보고자 하였다. 본 연구의 결과를 비추어볼 때, 시상면에서 평균 ASIA와 PSIA는 젊은 성인보다 고령자가 더 크게 나타났으며, 통계적으로 유의한 차이(각각 First Step의 ASIA, PSIA, Second Step의 ASIA, PSIA: p < 0.001, p = 0.023, p = 0.001, p < 0.001)가 있었다. ASIA와 PSIA의 값이 크다는 것은 두 가지 경우로 고려할 수 있는데, 첫 번째는 시상면에서의 최대 COM-COP의 경사각도가 감소(+y축의 감소)할 경우이다. 시상면의 최대 COM-COP의 경사각도는 Heel Strike와 Toe Off 지점에서 나타나기 때문에, 이는 보폭과 밀접한 관련이 있다. 두 번째는 입각기(Stance Phase)의 시간이 증가하여 한 발의 지지 시간이 증가(+x축의 증가)하는 경우를 고려할 수 있기 때문에, 보행속도와 밀접한 관련이 있다. 하지만, 시상면에서만 보행 안정성을 분석하기에는 한계점이 존재한다. 보행속도와 보폭은 운동학적 데이터에 많은 영향을 미치기 때문에, 보행 분석 시 잠재적인 낙상 가능성을 좀 더 정확히 파악하기 위해서는 보폭과 보행속도와 무관한 다른 방법이 필요하다. 보행 안정성은 이 외에 여러 가지 요인에 의하여 결정될 수 있지만, 그 중 기저면(Base of Support)에 대한 COM의 위치는 보행 안정성의 중요한 결정요인이다. 즉, COM이 기저면의 중심에 위치할 때 가장 안정적이며 중심에서 멀어질수록 보행 안정성은 약화된다. 보행은 전후 움직임이기 때문에, 전후보다는 좌우의 기저면이 짧으므로 관상면에서의 움직임을 같이 살펴보는 것이 보다 정확하게 보행 안정성을 측정할 수 있다고 생각된다. 그러므로, 관상면에서 최대 및 최소의 COM-COP 경사각도(Max FIA, Min FIA)를 나타냄으로써 보행 시 좌우로 벌어지는 경사각도를 통해 보행 안정성의 분석 방법을 보충하였다. 관상면에서의 평균 Max FIA와 Min FIA은 젊은 성인보다 고령자가 더 크게 나타났으며, 통계적으로 유의한 차이(각각 First Step의 Max FIA, Min FIA, Second Step의 Max FIA, Min FIA; p = 0.002, p = 0.011, p < 0.00001, p < 0.0001)가 있었다. 서론에서 한 예를 들어 말했듯이, 기존의 연구들에 의하면 고령자는 노화로 인하여 관절 경직도가 증가하거나, 균형 능력의 감소, 근력의 약화와 같은 신체적인 기능 상실이 발생하기 때문에 보행 안정성을 높이기 위한 보상 작용으로 보폭이 감소함으로써 입각기의 시간이 증가한다는 결과가 보고되었으며,4,5,24-26 건강한 80대 고령자는 20대의 건강한 대조군에 비해 걸음 간격(Step Width)과 두 발의 지지시간(Double Support Time)이 경미하게 증가하는 것으로 Bohannon 등에 의해 확인되었고,27 Greenspan 등은 일상 생활에서 고령자 낙상환자가 옆으로 넘어질 가능성이 높다고 보고되었다.28 이는 신체적인 기능 상실로 인해 상대적으로 고령자가 보행 시 옆으로 동작을 제어하는데 어려움이 있기 때문에, 관상면에서의 COM-COP 경사각도가 증가함이 나타날 수 있다. 결론적으로, 본 연구에서는 새로운 지표가 기존의 보행 안정성 연구 결과와 유사한 특징을 보였다. COM-COP 경사각도를 이용하여 기존 보행연구 결과의 특성과 유사하게 나타났다.
본 연구에서는 시상면의 ASIA, PSIA를 통해 SGSI를 도출하였으며, 관상면의 Max FIA, Min FIA를 통해 FGIA와 FGSI를 도출하여 젊은 성인과 고령자의 보행 대칭성을 비교하였으며, 보행 대칭성을 통해 보행 안정성을 분석하고자 하였다. Yoon 등에 의하면 고령자의 보행 훈련 기간이 증가할수록 시상면에서 최대 후방 COM-COP 경사각도가 증가하는 반면, 전방 COM-COP 경사각도는 감소한다고 보고되었다.29 또한 Lee 등의 연구에서는 건강한 고령자가 평형 기능에 문제가 있는 환자들에 비하여 시상면에서 최대 후방 COM-COP 경사각도가 증가한다고 보고되었으며,23 Hahn 등은 높은 장애물 위로 넘어갈 때 체간의 전방 흔들림이 커지기 때문에 COM이 상대적으로 더 앞쪽에 위치하므로 후방 COM-COP 경사각도가 증가한다고 보고하였다.30 그리고 Osoba 등은 보행의 변동성, 특히 좌우 변동의 증가가 고령자들에게 낙상 위험을 증가시킨다고 보고하였다.31 다른 선행연구에서는 연령과 BMI가 증가할수록 보행 시 동적 안정성을 확보하기 위해 보행속도와 보폭을 감소시킴과 함께 더 큰 걸음 간격을 가짐으로써 기저면을 증가시키는 결과가 보고되었다.27,28,32,33 따라서 본 연구에서는 젊은 성인과 고령자간 시상면에서는 보행 안정성의 차이가 없는 것으로 나타났다. 반면에 관상면에서는 고령자의 젊은 성인보다 관상면에서 FGIA가 더 크게 나타났기 때문에 고령자의 좌우 흔들림이 젊은 성인보다 크게 나타났을 것으로 보였다. 또한 BMI가 증가할수록 FGSI가 크게 나타났기 때문에 보행 시 고령자의 Second Step보다 First Step에서 보행 안정성이 감소한 것으로 나타났고, 특히 관상면에서 고령자의 경우 젊은 성인보다 BMI의 변화에 따라 보행 대칭성이 민감하게 변화하는 것으로 나타났다.
본 연구에서는 COM-COP 경사각도를 통해 보행 안정성을 분석하였으나, 몇 가지 한계점이 존재한다. 우선 첫 번째로, COM-COP 경사각도만으로는 보행 주기의 정확한 구간을 나눌 수 없었다. 따라서 본 연구에서는 시상면에서 볼 때 COM-COP 경사각도가 0o인 수직 지점을 Midstance로 설정하고, COP가 처음 측정되는 부분을 Heel Strike의 시작점, COP가 마지막으로 측정되는 부분을 Toe Off의 종료점으로 설정하였다. 두 번째로, 시상면에서 ASIA와 PSIA를 표현할 때 곡선을 직선회귀(R2 > 0.99)로 잡았다는 한계점을 갖는다. 세 번째, 시상면에서 ASIA와 PSIA를 60 Frame의 촬영속도를 통해 프레임 개수로 표현하였기 때문에, 다른 촬영속도로 분석할 경우, 이를 시간으로 환산해주어야 한다. 하지만, 본 연구의 목표는 COM-COP 경사각도를 통해 보행 안정성 판별이 가능한지를 확인하는 것이기 때문에 젊은 성인과 고령자의 상대적인 비교를 통한 보행 안정성 분석을 할 때에는 결과적으로 본 연구의 목표에 벗어나지 않았다고 사료된다. 네 번째로, 본 연구에서는 COM-COP 경사각도만을 이용했기 때문에, 보행 시 하지에 작용하는 힘에 대한 영향을 알 수 없었다. 또한 정확한 보행 구간을 알 수 없다 보니 보행 구간별 근육의 작용이나, 혹은 하지에 작용하는 힘의 영향으로 인해 COM과 COP의 진행을 결정하는 요인들에 대한 분석을 할 수 없었다. 마지막으로, 각 피험자들의 COM의 높이를 고려하지 않았다. 기존의 한 연구에서는 각 피험자의 COM의 높이의 차이에 따라 COM-COP 경사각도에 영향을 미칠 수도 있다고 가능성을 제안한 바 있다.34 하지만, 각 개인마다의 체형이 다르기 때문에 단순히 COM의 높이에 대한 변수만으로는 COM-COP 경사각도와의 관계를 정규화(Normalization)하는 것은 다소 부담스러울 것으로 판단한다.
본 연구에서는 COM-COP 경사각도의 새로운 지표를 통해 보행 안정성의 판별이 가능한지 확인하고자 하였으며, 젊은 성인과 고령자를 대상으로 정상체중, 과체중, 비만체중인 3가지의 BMI로 나누어 보행 안정성을 분석하기 위해 이를 검증해보고자 하였다. 결론적으로 본 연구에서도 본 연구에서는 COM-COP 경사각도의 새로운 지표가 기존의 보행 안정성 연구 결과와 유사한 특징을 보였으므로, COM-COP 경사각도의 새로운 지표를 통해 임상 적용 시 보다 쉽고 편하게 적용할 수 있으므로 사용할 만한 가치가 있다고 사료된다. 따라서 향후 보행 분석 시 보다 간소화된 분석 방법과 최소한의 데이터를 활용하여 보행 안정성을 분석할 수 있을 것으로 사료된다.
Acknowledgments
This research was supported by Basic Science Research Program through the National Research Foundation of Korea (NRF) funded by the Ministry of Education (No. NRF-2017R1D1A3B04033410).
REFERENCES
-
Sutherland, D. H., “The Evolution of Clinical Gait Analysis Part I: Kinesiological EMG,” Gait & Posture, Vol. 14, No. 1, pp. 61-70, 2001.
[https://doi.org/10.1016/S0966-6362(01)00100-X]
-
Sutherland, D. H., “The Evolution of Clinical Gait Analysis: Part II: Kinematics,” Gait & Posture, Vol. 16, No. 2, pp. 159-179, 2002.
[https://doi.org/10.1016/S0966-6362(02)00004-8]
-
Sutherland, D. H., “The Evolution of Clinical Gait Analysis Part III: Kinetics and Energy Assessment,” Gait & Posture, Vol. 21, No. 4, pp. 447-461, 2005.
[https://doi.org/10.1016/j.gaitpost.2004.07.008]
-
Cromwell, R. L. and Newton, R. A., “Relationship between Balance and Gait Stability in Healthy Older Adults,” Journal of Aging and Physical Activity, Vol. 12, No. 1, pp. 90-100, 2004.
[https://doi.org/10.1123/japa.12.1.90]
-
Hausdorff, J. M., Rios, D. A., and Edelberg, H. K., “Gait Variability and Fall Risk in Community-Living Older Adults: A 1-Year Prospective Study,” Archives of Physical Medicine and Rehabilitation, Vol. 82, No. 8, pp. 1050-1056, 2001.
[https://doi.org/10.1053/apmr.2001.24893]
- Abass, S., Jaffar, J., and Ghazi, M., “The Effects of Body Mass Index BMI on Human Gait Analysis,” The International Journal of Engineering and Science, Vol. 6, No. 10, pp. 46-54, 2017.
-
Lai, P. P., Leung, A. K., Li, A. N., and Zhang, M., “Three-Dimensional Gait Analysis of Obese Adults,” Clinical Biomechanics, Vol. 23, pp. 2-6, 2008.
[https://doi.org/10.1016/j.clinbiomech.2008.02.004]
-
Sarkar, A. and Sawhney, A., “Effects of Body Mass Index on Biomechanics of Adult Female Foot,” MOJ Anat & Physiol, Vol. 4, No. 1, pp. 232-236, 2017.
[https://doi.org/10.15406/mojap.2017.04.00124]
-
Ko, S. U., Ling, S. M., Schreiber, C., Nesbitt, M., and Ferrucci, L., “Gait Patterns during Different Walking Conditions in Older Adults with and without Knee Osteoarthritis-Results from the Baltimore Longitudinal Study of Aging,” Gait & Posture, Vol. 33, No. 2, pp. 205-210, 2011.
[https://doi.org/10.1016/j.gaitpost.2010.11.006]
- Abbass, S. J. and Abdulrahman, G., “Kinematic Analysis of Human Gait Cycle,” Al-Nahrain Journal for Engineering Sciences, Vol. 16, No. 2, pp. 208-222, 2013.
-
Nor, M. M., Zakaria, N. K., Jailani, R., and Tahir, N., “Analysis of EMG Signals during Walking of Healthy Children,” Procedia Computer Science, Vol. 76, pp. 316-322, 2015.
[https://doi.org/10.1016/j.procs.2015.12.299]
-
Horstmann, T., Listringhaus, R., Haase, G. B., Grau, S., and Mündermann, A., “Changes in Gait Patterns and Muscle Activity Following Total Hip Arthroplasty: A Six-Month Follow-up,” Clinical Biomechanics, Vol. 28, No. 7, pp. 762-769, 2013.
[https://doi.org/10.1016/j.clinbiomech.2013.07.001]
-
Collins, J. J. and De Luca, C. J., “Open-Loop and Closed-Loop Control of Posture: A Random-Walk Analysis of Center-of-Pressure Trajectories,” Experimental Brain Research, Vol. 95, No. 2, pp. 308-318, 1993.
[https://doi.org/10.1007/BF00229788]
- Goldie, P. A., Bach, T., and Evans, O., “Force Platform Measures for Evaluating Postural Control: Reliability and Validity,” Archives of Physical Medicine and Rehabilitation, Vol. 70, No. 7, pp. 510-517, 1989.
-
Pai, Y. C. and Patton, J., “Center of Mass Velocity-Position Predictions for Balance Control,” Journal of Biomechanics, Vol. 30, No. 4, pp. 347-354, 1997.
[https://doi.org/10.1016/S0021-9290(96)00165-0]
-
Yamaguchi, T. and Masani, K., “Contribution of Center of Mass-Center of Pressure Angle Tangent to the Required Coefficient of Friction in the Sagittal Plane during Straight Walking,” Biotribology, Vol. 5, pp. 16-22, 2016.
[https://doi.org/10.1016/j.biotri.2015.12.002]
-
Meurisse, G. M., Bastien, G. J., and Schepens, B., “Effect of Age and Speed on the Step-to-Step Transition Phase during Walking,” Journal of Biomechanics, Vol. 83, pp. 253-259, 2019.
[https://doi.org/10.1016/j.jbiomech.2018.12.001]
-
Krebs, D. E., McGibbon, C. A., and Goldvasser, D., “Analysis of Postural Perturbation Responses,” IEEE Transactions on Neural Systems and Rehabilitation Engineering, Vol. 9, No. 1, pp. 76-80, 2001.
[https://doi.org/10.1109/7333.918279]
-
Xu, R., Wang, X., Yang, J., He, F., Zhao, X., et al., “Comparison of the COM-FCP Inclination Angle and Other Mediolateral Stability Indicators for Turning,” Biomedical Engineering Online, Vol. 16, No. 1, pp. 1-15, 2017.
[https://doi.org/10.1186/s12938-017-0325-z]
-
Huang, S. C., Lu, T. W., Chen, H. L., Wang, T. M., and Chou, L. S., “Age and Height Effects on the Center of Mass and Center of Pressure Inclination Angles during Obstacle-Crossing,” Medical Engineering & Physics, Vol. 30, No. 8, pp. 968-975, 2008.
[https://doi.org/10.1016/j.medengphy.2007.12.005]
-
Hong, S. W., Leu, T. H., Wang, T. M., Li, J. D., Ho, W. P., et al., “Control of Body's Center of Mass Motion Relative to Center of Pressure during Uphill Walking in the Elderly,” Gait & Posture, Vol. 42, No. 4, pp. 523-528, 2015.
[https://doi.org/10.1016/j.gaitpost.2015.08.007]
-
Wang, T. M., Chen, H. L., Hsu, W. C., Liu, M. W., and Lu, T. W., “Biomechanical Role of the Locomotor System in Controlling Body Center of Mass Motion in Older Adults during Obstructed Gait,” Journal of Mechanics, Vol. 26, No. 2, pp. 195-203, 2010.
[https://doi.org/10.1017/S1727719100003051]
-
Lee, H. J. and Chou, L. S., “Detection of Gait Instability Using the Center of Mass and Center of Pressure Inclination Angles,” Archives of Physical Medicine and Rehabilitation, Vol. 87, No. 4, pp. 569-575, 2006.
[https://doi.org/10.1016/j.apmr.2005.11.033]
-
Kerrigan, D. C., Todd, M. K., Della Croce, U., Lipsitz, L. A., and Collins, J. J., “Biomechanical Gait Alterations Independent of Speed in the Healthy Elderly: Evidence for Specific Limiting Impairments,” Archives of Physical Medicine and Rehabilitation, Vol. 79, No. 3, pp. 317-322, 1998.
[https://doi.org/10.1016/S0003-9993(98)90013-2]
- Öberg, T., Karsznia, A., and Öberg, K., “Basic Gait Parameters: Reference Data for Normal Subjects, 10-79 Years of Age,” Journal of Rehabilitation Research and Development, Vol. 30, pp. 210-210, 1993.
-
Winter, D. A., Patla, A. E., Frank, J. S., and Walt, S. E., “Biomechanical Walking Pattern Changes in the Fit and Healthy Elderly,” Physical Therapy, Vol. 70, No. 6, pp. 340-347, 1990.
[https://doi.org/10.1093/ptj/70.6.340]
-
Bohannon, R. W., “Comfortable and Maximum Walking Speed of Adults Aged 20-79 Years: Reference Values and Determinants,” Age and Ageing, Vol. 26, No. 1, pp. 15-19, 1997.
[https://doi.org/10.1093/ageing/26.1.15]
-
Greenspan, S. L., Myers, E. R., Kiel, D. P., Parker, R. A., Hayes, W. C., et al., “Fall Direction, Bone Mineral Density, and Function: Risk Factors for Hip Fracture in Frail Nursing Home Elderly,” The American Journal of Medicine, Vol. 104, No. 6, pp. 539-545, 1998.
[https://doi.org/10.1016/S0002-9343(98)00115-6]
-
Yoon, S. H., Kim, T. S., Lee, J. H., Ryu, J. S., and Kwon, Y. H., “Evaluation of the Elderly Gait Stability Using the Center of Mass and Center of Pressure Inclination Angles,” Korean Journal of Sport Biomechanics, Vol. 17, No. 4, pp. 99-106, 2007.
[https://doi.org/10.5103/KJSB.2007.17.4.099]
-
Hahn, M. E. and Chou, L. S., “Age-Related Reduction in Sagittal Plane Center of Mass Motion during Obstacle Crossing,” Journal of Biomechanics, Vol. 37, No. 6, pp. 837-844, 2004.
[https://doi.org/10.1016/j.jbiomech.2003.11.010]
-
Osoba, M. Y., Rao, A. K., Agrawal, S. K., and Lalwani, A. K., “Balance and Gait in the Elderly: A Contemporary Review,” Laryngoscope Investigative Otolaryngology, Vol. 4, No. 1, pp. 143-153, 2019.
[https://doi.org/10.1002/lio2.252]
-
Hills, A. P. and Parker, A. W., “Gait Characteristics of Obese Children,” Archives of Physical Medicine and Rehabilitation, Vol. 72, No. 6, pp. 403-407, 1991.
[https://doi.org/10.1097/01241398-199111000-00032]
-
Browning, R. C. and Kram, R., “Effects of Obesity on the Biomechanics of Walking at Different Speeds,” Medicine & Science in Sports & Exercise, Vol. 39, No. 9, pp. 1632-1641, 2007.
[https://doi.org/10.1249/mss.0b013e318076b54b]
-
Berger, W., Trippel, M., Discher, M., and Dietz, V., “Influence of Subjects’ Height on the Stabilization of Posture,” Acta Oto-laryngologica, Vol. 112, No. 1, pp. 22-30, 1992.
[https://doi.org/10.3109/00016489209100778]
-
Hamacher, D., Singh, N., Van Dieën, J. H., Heller, M., and Taylor, W. R., “Kinematic Measures for Assessing Gait Stability in Elderly Individuals: A Systematic Review,” Journal of the Royal Society Interface, Vol. 8, No. 65, pp. 1682-1698, 2011.
[https://doi.org/10.1098/rsif.2011.0416]
-
Hamacher, D., Liebl, D., Hödl, C., Heßler, V., Kniewasser, C. K., et al., “Gait Stability and Its Influencing Factors in Older Adults,” Frontiers in Physiology, Vol. 9, Paper No. 1955, 2019.
[https://doi.org/10.3389/fphys.2018.01955]
-
Bruijn, S. M., Meijer, O., Beek, P., and van Dieen, J. H., “Assessing the Stability of Human Locomotion: A Review of Current Measures,” Journal of the Royal Society Interface, Vol. 10, No. 83, Paper No. 20120999, 2013.
[https://doi.org/10.1098/rsif.2012.0999]
-
Bisi, M. C., Riva, F., and Stagni, R., “Measures of Gait Stability: Performance on Adults and Toddlers at the Beginning of Independent Walking,” Journal of NeuroEngineering and Rehabilitation, Vol. 11, No. 1, pp. 1-9, 2014.
[https://doi.org/10.1186/1743-0003-11-131]
-
Gallagher, S., Kotowski, S., Davis, K. G., Mark, C., Compton, C. S., et al., “External L5-S1 Joint Moments When Lifting Wire Mesh Screen Used to Prevent Rock Falls in Underground Mines,” International Journal of Industrial Ergonomics, Vol. 39, No. 5, pp. 828-834, 2009.
[https://doi.org/10.1016/j.ergon.2009.01.005]
Master Candidate in the Department of Convergence Engineering, Jungwon University in Korea. His research interest is the computational mechanics related to dynamic analysis.
E-mail: a01032227634@gmail.com
Associate Professor in the Department of Biomedical Engineering, Jungwon University in Korea. His research interests include musculoskeletal dynamics and surgical navigation in orthopedic & rehabilitation research and other physics-based simulations related to biomedical devices.
E-mail: bmebae@jwu.ac.kr