
미세 입자 분리를 위한 유체 채널 내 폴리머 벽을 포함한 음파영동 디바이스의 제작
Copyright © The Korean Society for Precision Engineering
This is an Open-Access article distributed under the terms of the Creative Commons Attribution Non-Commercial License (http://creativecommons.org/licenses/by-nc/3.0) which permits unrestricted non-commercial use, distribution, and reproduction in any medium, provided the original work is properly cited.
Abstract
In this paper, we propose acoustophoretic microfluidic devices with an acoustic transparent polymer wall using a simple and low-cost fabrication method followed by MEMS (Micro-Electromechanical Systems) processes. Generally, due to the acoustic standing wave between two opposing walls in microfluidic channel, the particle focusing lines are fixed according to the applied frequency. In the proposed device, however, it is possible to place the particle focusing lines in the arbitrary position within the fluidic domain through the optimized width of polymer wall. The PDMS (Polydimethylsiloxane) mold with thin layer was used as the sealing layer between the Si substrate and cover glass, as well as the decoupling layer between the acoustic boundary and fluidic boundary. The thickness of PDMS mold needed to be minimized to decrease the heating by the acoustic energy absorption of PDMS layer, which was successfully made using the spin-coating of PDMS and the UV tape transfer method. The acoustophoretic device with thin PDMS layer and optimized width of PDMS wall can be applied, for biotechnological applications such as the separation of blood cells and micro-particles.
Keywords:
Acoustophoretic device, Acoustic standing wave, Acoustic attenuation, Particle separation, Polymer wall, PDMS molding키워드:
음파영동 디바이스, 정상음향파, 음파 감쇠, 입자 분리, 폴리머 벽, PDMS 몰딩1. 서론
최근 소량의 시료를 분리하기 위한 마이크로 스케일의 다양한 입자 분리 방법들이 소개되고 있다. 그 중에서도 미세유체역학(Microfluidics)을 기반으로 하는 많은 연구가 진행되고 있는데, 대표적으로 전기영동(Electrophoresis) [1,2], 자기영동(Magnetophoresis) [3], 음파영동(acoustophoresis) [4-15] 등이 있다. 특히, 음파영동기술은 전기영동, 자기영동 기술에 비해 세포와 같은 생체 시료 분리에 있어 생체적합성(Biocompatibility)이 뛰어날 뿐만 아니라 간단한 구조의 디바이스에서 대량으로 입자 분리가 가능하다는 장점을 가지고 있다. 이에 따라 생물학적 시료 분석 분야에서 음파영동을 이용한 혈액 및 세포의 조작과 세척 등의 응용 연구가 활발히 진행되고 있다[8-12,15].
음파영동기술은 표면탄성파(Surface Acoustic Wave) [4,5] 또는 벌크탄성파(Bulk Acoustic Wave) [5-12]를 이용하여 입자를 분리하는 기술로, 특히 미세 채널 내에서의 정상음향파(Acoustic Standing Wave)는 입자들을 Pressure Node 또는 Pressure Anti-Node로 움직이게 할 수 있다. 이와 같이 유체 채널 내에서 벌크 탄성파를 이용한 연속적인 입자 분리를 위하여 여러 다양한 미세유체 디바이스들이 제작되었다. 그러나 이를 이용한 입자 분리는 Pressure Node 및 Anti-Node가 아닌 위치에서의 입자 집중이 불가하다는 한계를 가지고 있다. 따라서 미세유체 채널 내의 임의의 위치에서 입자 집중을 가능하게 하는 연구가 필요하다. 이를 위해 Pressure Node 형성에 영향을 주는 음파경계층과 유체가 흐르는 유체경계층을 분리하는 방법을 통해 이 문제를 해결하고자 하였다.
Fong, et al. [13]은 에코 채널(Echo Channel)과 메인 채널(Main Channel) 사이에 얇은 실리콘 벽을 가지는 디바이스를 제작하고, 이를 이용하여 임의의 위치에 입자를 분리하는 방법을 제안하였다. 이 방법은 에코 채널 내 유체의 물성에 따라 Pressure Node가 만들어지는 위치를 조절할 수 있어 메인 채널 내의 임의의 위치에 입자를 집중시킬 수 있었다. 그러나 에코 채널과 메인 채널 사이에 얇은 실리콘 벽을 가지는 디바이스를 제작하기 위해서 deep-RIE (Reactive Ion Etching)와 같은 고가의 장비를 이용하여 해야 하는 어려움이 있다.
한편, Leibacher, et al. [14]은 미세 채널 내에 음파투과층(Acoustic Transparent Layer)을 위치시켜 고정된 위치가 아닌 원하는 위치에서 입자를 분리할 수 있는 방법을 제안하였다. 이들은 음파투과층으로서 PDMS (Polydimethylsiloxane)를 이용하였고, 이를 채널 내의 원하는 곳에 위치시키기 위해 레이저를 이용하여 가공하였다. PDMS는 미세유체역학 및 랩온어칩(Lab-on-a-Chip) 분야에서 널리 사용되며 생체적합성이 매우 뛰어날 뿐만 아니라, 특히 물(Water)과의 음향 임피던스(Acoustic Impedance)가 유사하여 음파투과층으로서 음파영동 디바이스 제작에 적합한 재료이다. 그러나 레이저를 이용한 방법은 높은 정확성을 가진다는 이점이 있는 반면, 채널 크기에 제한이 발생하며, 커팅 시 발생하는 열에 의해 PDMS 층의 변형이 발생하여 수직 형태의 PDMS 벽(Wall)을 제작하는 데에는 어려움이 생길 수 있다.
본 연구에서는 기본적인 MEMS (Microelectro-Mechanical Systems) 공정인 포토리소그라피(Photo-Lithography) 및 실리콘 이방성 습식 식각(Anisotropic Wet Etching), 플라즈마 본딩(Plasma Bonding) 공정 등을 이용하여 기존 방법에 비해 간단하게 미세 채널 내에 PDMS 벽이 있는 음파영동 디바이스를 제작할 수 있는 방법을 제안한다. 본 연구에서 제안한 제작 방법은 기존의 제작 방법[14]에 비해 레이저 가공 시 발생하는 PDMS 벽의 변형에 의한 불균일성과 같은 문제를 해결할 수 있을 뿐아니라 미세 채널의 폭과 깊이를 조절하는 것이 자유로우며 특히 깊은 채널의 제작에도 적용이 가능하다. 또한, PDMS 층의 두께가 두꺼울수록 음파 감쇠(Acoustic Attenuation)가 커지게 되어 음파영동 디바이스의 효율이 떨어지게 되는데[16], 본 연구에서 제안한 방법은 PDMS 벽을 포함한 PDMS 층의 두께를 얇고 균일하게 제작할 수 있어 음파 감쇠에 따른 발열 현상을 최소화할 수 있을 뿐 아니라 PDMS 층과 실리콘 채널, 커버 글라스(Cover Glass)와의 밀착된 본딩이 가능하다. 이와 같이 제작된 디바이스를 이용하여 미세 채널 내부에서 발생하는 미세입자의 집중 현상을 관찰하였다.
2. 본론
Fig. 1은 음파영동 미세유체 채널과 그 속에서 정상음향파에 의해 생성되는 Pressure Node를 나타낸다. 이때 Fig. 1(b)는 음파투과층을 이용하여 음파경계층과 유체경계층을 분리(Decoupling)시켜 미세유체 채널 내의 임의의 지점에 Pressure Node를 형성할 수 있음을 보여주고 있다.
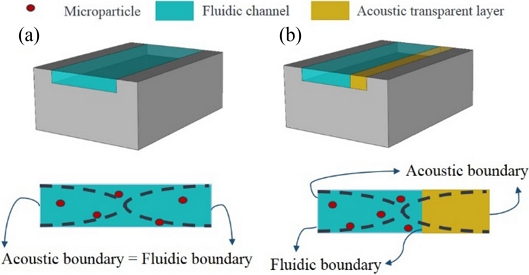
Illustration of relation between acoustic boundary and fluidic boundary across a microfluidic channel. (a) Coupled state, and (b) Decoupled state by acoustic transparent layer
본 연구에서는 PDMS를 음파투과층으로 이용하여 음파경계층과 유체경계층이 분리된 음파영동 미세유체 디바이스를 제작하기 위해 Fig. 2에서와 같이 포토 리소그라피, 실리콘 이방성 습식 식각 및 플라즈마 본딩 공정을 순차적으로 진행하였다. 먼저 포토리소그라피 공정을 위하여 500 nm 두께의 실리콘 산화막(SiO2)층이 증착된 4인치 (100)단 결정 실리콘 웨이퍼를 이용하였다. 양성 감광제(Positive Photoresist)인 AZ 601을 1 μm 두께로 스핀 코팅(Spin-Coating)한 후 100oC에서 60초간 소프트 베이크(Soft Bake)를 진행하였다. 이후 노광(Exposure) 공정과 현상(Develop) 공정을 진행하여 채널 패턴을 형성하였다. 이때 실리콘 이방성 습식식각을 이용하여 음파가 진행하는 채널의 양쪽 벽면을 수직으로 제작하기 위해 포토마스크(Photomask)의 채널 방향과 (100) 웨이퍼의 플랫면이 45o를 이루도록 정렬(Align)을 하였다. BOE (Buffered Oxide Etchant) 용액을 이용하여 실리콘 산화막 층을 식각하였다(Fig. 2(a)). 이후 남아있는 감광제는 아세톤 용액과 IPA (Isopropyl Alcohol) 용액을 이용하여 제거하였다. 산화막이 패터닝된 웨이퍼에 대해 수산화칼륨(KOH, Potassium Hydroxide) 용액을 이용하여 실리콘 이방성 습식 식각을 수행하였다(Fig. 2(b)). 사용된 KOH 용액의 농도는 30%, 온도는 80o로, 이때의 식각 속도는 약 1.2 μm/min로 측정되었다. 이를 통해 실리콘 웨이퍼 위에 미세유체 채널과 PDMS 몰드용 실리콘 마스터 몰드를 형성한 후 식각 마스크로 이용되었던 실리콘 산화막 층은 BOE 용액을 이용하여 제거하고, 다이싱(Dicing) 공정을 통해 실리콘 웨이퍼상에 함께 형성된 실리콘 미세유체 채널과 실리콘 마스터 몰드를 분리하였다(Fig. 2(c)). 분리된 실리콘 마스터 몰드로부터 PDMS 몰드를 제작한 후(Fig. 2(d)), 실리콘 채널과 PDMS 몰드의 본딩을 위해 O2 플라즈마 처리를 실시하였다. 이때 PDMS 몰드의 PDMS 벽이 실리콘 채널의 가장자리에 정확하게 정렬이 되도록 두 기판 사이에 약간의 물을 첨가한 후 본딩을 진행하였다[17,18] (Fig. 2(e)). 이후 두 기판 사이의 물이 모두 증발되면 PDMS 몰드의 PDMS층과 커버 글라스의 본딩을 위해 다시 한 번 더 O2 플라즈마 처리를 수행하여 최종 디바이스를 완성하였다(Fig. 2(f)).
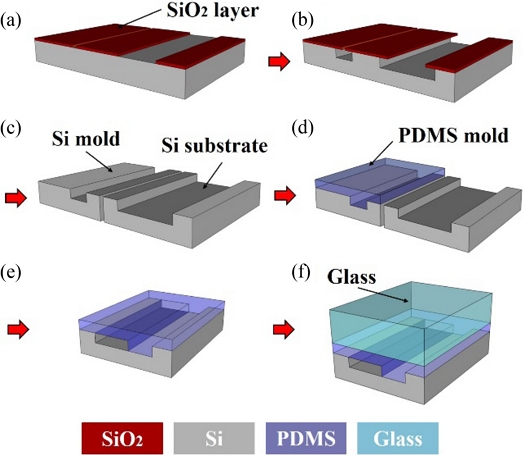
Schematic view of fabrication processes of acoustophoretic device with lateral PDMS wall. (a) SiO2 layer patterning, (b) Si wet etching, (c) SiO2 layer removal and dicing, (d) PDMS molding, (e) 1st plasma bonding between Si substrate and PDMS mold, and (f) 2nd plasma bonding between PDMS and cover glass
Fig. 3은 PDMS 몰딩 공정(Fig. 2(d)) 및 두 단계의 플라즈마 본딩 공정(Figs. 2(e)와 2(f))에 대해 보다 얇은 두께를 가지며 PDMS 몰드의 변형을 최소화할 수 있는 제작 공정을 나타낸다. 스핀 코터(Spin-Coater)의 회전속도(rpm)에 따라 PDMS 몰드의 두께를 조절하였으며, Table 1은 실험을 통해 구한 스핀 코터의 회전속도 및 시간과 PDMS 두께의 관계를 나타낸다. Fig. 3(b)에서와 같이 실리콘 몰드로부터 얇은 PDMS 몰드를 변형 없이 분리하기 위하여 UV 테이프를 이용하였다[19]. 이를 통해 실리콘 몰드와 분리하는 과정에서 PDMS의 변형을 최소화할 수 있었으며, PDMS 층과 PDMS 벽이 내재된 음파영동 디바이스를 성공적으로 제작할 수 있었다.
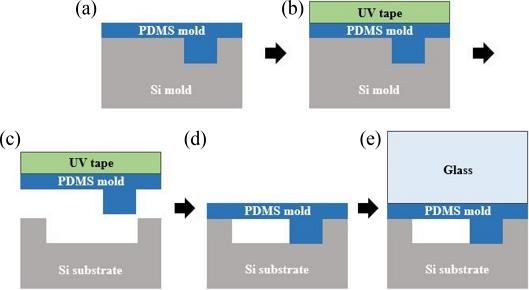
Schematic view of fabrication processes of thin PDMS layer. (a) PDMS molding using spin-coating, (b) UV tape attachment to PDMS mold, (c) PDMS mold detachment from Si mold, (d) 1st plasma bonding between Si substrate and PDMS mold, and (e) 2nd plasma bonding between PDMS and cover glass
Fig. 4는 음파영동을 이용한 입자 집중 실험을 위한 장치의 구성도 및 실제 사용된 장치의 사진을 보여준다. 음파영동을 이용한 입자 집중 실험에서 Pressure Node가 생성되는 지점은 가해진 전압의 주파수와 채널 폭 사이의 관계에서 구할 수 있다. 이전 연구[20]에 따르면 제작된 디바이스의 미세 채널 폭이 375 μm, 물 속에서의 음파의 속력(20oC)을 대략 1,500 m/s라고 한다면 가해진 전압의 주파수가 약 2 MHz (Fundamental Resonance Frequency)일 때 첫 번째 정상음향파의 Pressure Node가 미세 채널 중앙에 형성된다. 본 연구에서는 첫 번째 정상음향파를 이용하여 입자 집중 현상을 확인하기 위해 함수발생기를 이용하여 약 1.93 MHz의 사인파(Sinusoidal Wave)를 전력 증폭기(Power Amplifier)를 통해 디바이스 하단에 부착된 압전소자(Piezoelectric Transducer, PZT)에 인가하였다. 또한, 입자 집중 현상이 발생하는 때의 인가 전압을 확인하기 위하여 전력 증폭기에 오실로스코프(Oscilloscope)를 연결하였으며, 이때 압전소자에 인가된 전압은 20-30 V이었다. 한편, 음파영동 디바이스의 온도를 일정하게 유지하기 위하여 펠티어(Peltier) 소자를 이용한 냉각 시스템(Cooling System)을 압전소자 하단에 부착하였다[6-9].
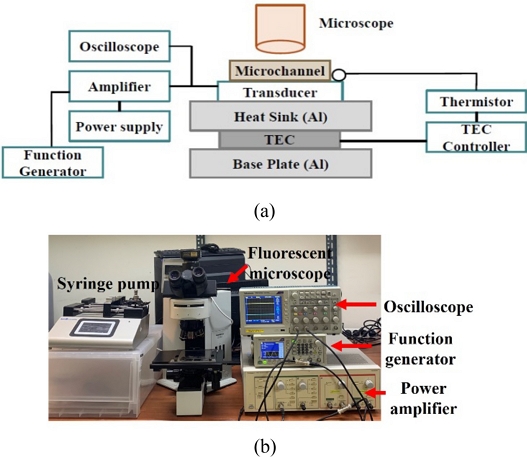
(a) Schematic view, and (b) Actual equipment of experimental setup for particle separation experiment
실험에 사용된 입자는 직경 10 μm의 폴리스티렌 입자(Polybead Polystyrene 10.0 Micron Microsphere, Polyscience Inc.)로 탈이온수(DI Water)에 1 : 25의 비율로 희석하여 사용하였다. 시린지 펌프(LEGATO 111, KD Scientific Inc.)를 통해 1 ml/hr의 속도로 유체를 흘려주었으며, 입자의 집중 여부는 광학현미경(BX-60, Olympus)과 디지털 CMOS 카메라(Touptek Photonics Co., Ltd.)를 통해 관찰하였다.
3. 실험 결과 및 고찰
본 연구에서는 정해진 위치가 아닌 원하는 위치에서의 입자 집중을 위해 PDMS 벽을 포함한 음파영동 미세유체 디바이스를 제작하였다. Fig. 5는 미세유체 채널 단면의 주사전자 현미경(Scanning Electron Microscope, SEM) 사진으로 스핀 코팅을 사용하지 않고, 일반적인 PDMS 몰딩법으로 제작되었다. 음파영동 디바이스의 유체경계층의 채널 폭은 375, 깊이는 55-60 μm이며, PDMS 몰드의 폭은 150 μm로 유체경계층을 형성하였다. 이때 PDMS 층의 두께는 250, 1,000 μm로, PDMS를 마스터 몰드에 부어 자체 중력을 이용하는 일반적인 PDMS 몰딩법의 경우 표면장력 등의 영향으로 두께에 차이가 생기기 쉬우며, 바닥이 편평하지 않은 경우 경사지게 되어 커버 글라스와의 밀착된 본딩이 곤란한 경우가 발생하였다. 또한, PDMS가 얇아지게 되면 정전기 등의 영향으로 핸들링이 어려워 정렬 불량(Misalignment)이 발생하거나 PDMS 층이 실리콘 채널과 균일하게 밀착된 본딩이 이루어지지 않아 누수(Leakage)가 발생하였다. 반면, Fig. 6에서와 같이 PDMS 몰딩 시 스핀 코팅을 이용한 경우에는 PDMS 층의 두께를 쉽게 조절할 수 있었으며, 반도체 패키징 공정에서 자주 사용되는 UV 테이프를 이용하여 보다 얇고 균일한 PDMS 층을 손쉽게 실리콘 채널과 정렬 후 본딩을 진행할 수 있었다.
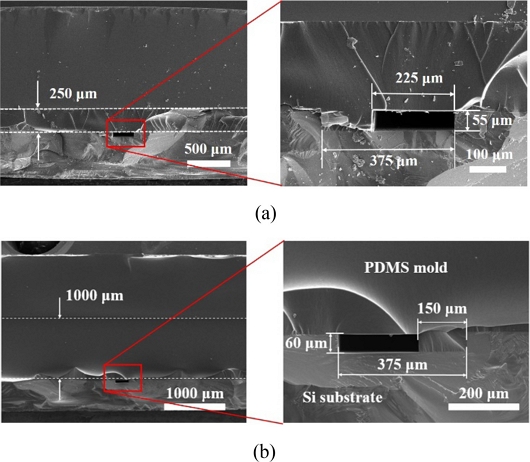
Cross-sectional SEM images of acoustophoretic channel without using spin-coating. The PDMS layer thickness is (a) 250, and (b) 1,000 μm
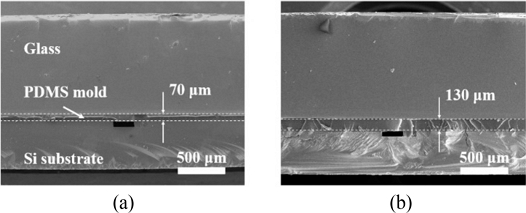
Cross-sectional SEM images of acoustophoretic channel using spin-coating. The PDMS layer thickness is (a) 70, and (b) 130 μm
이와 같이 제작된 음파영동 디바이스의 하단부에 압전소자를 부착한 후 전압을 인가하여 입자 집중 실험을 진행하였다(Fig. 7). 함수발생기에서 발생하는 사인파의 주파수를 적절히 조절하여 정상음향파가 만들어진 경우 Fig. 7(b)와 같이 정상음향파의 Pressure Node 부근에 입자가 집중되는 현상을 나타내었다. 유체 채널 경계면을 기준으로 보았을 때 기존의 PDMS 벽이 없는 음파영동 디바이스에서와 달리 유체 채널의 중앙이 아닌 임의의 위치에 입자가 집중되는 효과가 발생함을 알 수 있다.
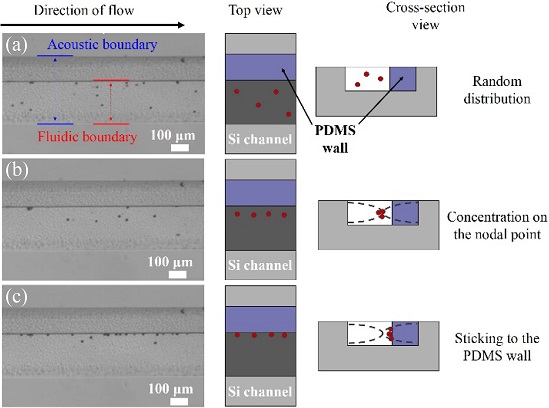
Microscopic images and schematic views of polystyrene particles migrated. (a) Randomly distributed particles without applied acoustic standing wave, (b) Focused particles on nodal point with low power acoustic standing wave, and (c) Particles stick to PDMS wall with high power acoustic standing wave (Blue line: Acoustic boundary, Red line: Fluidic boundary)
다양한 두께의 PDMS 층을 가진 디바이스에 대해 입자 집중 실험을 진행한 결과, PDMS 층의 두께가 두꺼울수록 음파 감쇠가 커져 이를 보상하기 위해서 입자 집중 실험 시 더욱 높은 전압을 인가하는 것이 필요하였다[16]. 이와 같이 인가전압이 높아질수록 Fig. 7(c)와 같이 입자들이 PDMS 벽에 달라붙는 Sticking 현상이 자주 발생하였다. 이러한 현상들은 앞에서도 언급한 바와 같이 일반적인 PDMS 몰딩법을 이용하여 제작한 디바이스에서 자주 발생한 반면 PDMS 층의 두께가 얇을수록 낮은 전압에서 Sticking 현상 없이 정상적으로 입자 집중이 이루어졌다. 이로부터 PDMS 벽과 PDMS 층에 의한 음파 감쇠로 인한 음파 에너지 소실이 Sticking 현상들에 영향을 미치는 것으로 판단된다. 즉, PDMS에 흡수된 에너지로 인해 정상음향파에 의한 Pressure Node에서의 음향방사력(Acoustic Radiation Force)가 감소하게 되어 입자 집중을 위해 더 높은 인가전압이 필요로 하게 되는데, 이로 인해 입자들의 Sticking 현상이 보다 쉽게 발생한 것으로 판단된다. 따라서, PDMS 몰딩 시 스핀 코팅과 UV 테이프를 이용하는 것은 PDMS 벽을 가지는 음파영동 디바이스 제작에 있어 중요한 문제 해결 방안으로 활용될 수 있을 것으로 기대된다.
4. 결론
본 연구에서는 기본적인 MEMS 공정을 이용하여 PDMS 벽을 포함한 음파영동 미세유체 디바이스의 제작 방법을 제안하였다. PDMS 몰딩 시 스핀 코팅과 UV 테이프를 이용하여 PDMS 벽을 포함한 PDMS 몰드를 얇게 제작할 수 있었다. 이를 통해 낮은 인가전압에서 디바이스를 작동하여 디바이스의 발열을 줄일 수 있었을 뿐 아니라 실리콘 채널 및 커버 글라스와의 플라즈마 본딩 과정 시 발생하는 누수 및 정렬 불량 현상도 최소화할 수 있었다. 음파경계층과 유체경계층이 분리된 디바이스를 이용하여 미세 채널 내 임의의 위치에서 형성되는 Pressure Node를 따라 미세 입자들이 집중됨을 확인하였다. 본 연구에서 개발된 음파영동 미세유체 디바이스는 세포 계수 및 분리와 같은 바이오 분야에서 활용될 수 있을 것으로 기대된다.
Acknowledgments
이 연구는 서울과학기술대학교 교내 학술연구비 지원으로 수행되었습니다.
REFERENCES
-
Ou, X., Chen, P., Huang, X., Li, S., Liu, B. F., (2020), Microfluidic chip electrophoresis for biochemical analysis, Journal of Separation Science, 43(1), 258-270.
[https://doi.org/10.1002/jssc.201900758]
-
Kohlheyer, D., Eijkel, J. C., van den Berg, A., Schasfoort, R. B., (2008), Miniaturizing free-flow electrophoresis-a critical review, Electrophoresis, 29(5), 977-993.
[https://doi.org/10.1002/elps.200700725]
-
Zborowski, M., Ostera, G. R., Moore, L. R., Milliron, S., Chalmers, J. J., Schechter, A. N., (2003), Red blood cell magnetophoresis, Biophysical Journal, 84(4), 2638-2645.
[https://doi.org/10.1016/S0006-3495(03)75069-3]
-
Lin, S.-C. S., Mao, X., Huang, T. J., (2012), Surface acoustic wave (SAW) acoustophoresis: Now and beyond, Lab on a Chip, 12(16), 2766-2770.
[https://doi.org/10.1039/c2lc90076a]
-
Chen, Y., Nawaz, A. A., Zhao, Y., Huang, P.-H., McCoy, J. P., Levine, S. J., Wang, L., Huang, T. J., (2014), Standing surface acoustic wave (SSAW)-based microfluidic cytometer, Lab on a Chip, 14(5), 916-923.
[https://doi.org/10.1039/C3LC51139A]
-
Wiklund, M., (2012), Acoustofluidics 12: Biocompatibility and cell viability in microfluidic acoustic resonators, Lab on a Chip, 12(11), 2018-2028.
[https://doi.org/10.1039/c2lc40201g]
-
Lenshof, A., Laurell, T., (2011), Emerging clinical applications of microchip-based acoustophoresis, Journal of the Association for Laboratory Automation, 16(6), 443-449.
[https://doi.org/10.1016/j.jala.2011.07.004]
- Nam, J., Lim, H., Kim, D., Shin, S., (2011), Acoustic device for selective platelet extraction from whole blood, Proceedings of the 15th International Conference on Miniaturized Systems for Chemistry and Life Sciences 2011, 1326-1328.
-
Petersson, F., Nilsson, A., Holm, C., Jönsson, H., Laurell, T., (2005), Continuous separation of lipid particles from erythrocytes by means of laminar flow and acoustic standing wave forces, Lab on a Chip, 5(1), 20-22.
[https://doi.org/10.1039/B405748C]
- Doria, A., Martin, N. E., Lee, A. P., (2012), Rapid two-step blood sample preparation with acoustic microfluidic chips, Proceedings of the 16th International Conference on Miniaturized Systems for Chemistry and Life Sciences, 1366-1368.
-
Laurell, T., Petersson, F., Nilsson, A., (2007), Chip integrated strategies for acoustic separation and manipulation of cells and particles, Chemical Society Reviews, 36(3), 492-506.
[https://doi.org/10.1039/B601326K]
-
Grenvall, C., Magnusson, C., Lilja, H., Laurell, T., (2015), Concurrent isolation of lymphocytes and granulocytes using prefocused free flow acoustophoresis, Analytical Chemistry, 87(11), 5596-5604.
[https://doi.org/10.1021/acs.analchem.5b00370]
-
Fong, E. J., Johnston, A. C., Notton, T., Jung, S.-Y., Rose, K. A., Weinberger, L. S., Shusteff, M., (2014), Acoustic focusing with engineered node locations for high-performance microfluidic particle separation, Analyst, 139(5), 1192-1200.
[https://doi.org/10.1039/C4AN00034J]
-
Leibacher, I., Schatzer, S., Dual, J., (2014), Impedance matched channel walls in acoustofluidic systems, Lab on a Chip, 14(3), 463-470.
[https://doi.org/10.1039/C3LC51109J]
-
Lissandrello, C., Dubay, R., Kotz, K. T., Fiering, J., (2018), Purification of lymphocytes by acoustic separation in plastic microchannels, SLAS Technology: Translating Life Sciences Innovation, 23(4), 352-363.
[https://doi.org/10.1177/2472630317749944]
-
Shu, X., Liu, H., Zhu, Y., Cai, B., Jin, Y., Wei, Y., Zhou, F., Liu, W., Guo, S., (2018), An improved bulk acoustic waves chip based on a PDMS bonding layer for high-efficient particle enrichment, Microfluidics and Nanofluidics, 22(3), 1-7.
[https://doi.org/10.1007/s10404-018-2052-6]
-
Truxal, S. C., Tung, Y.-C., Kurabayashi, K., (2008), A flexible nanograting integrated onto silicon micromachines by soft lithographic replica molding and assembly, Journal of Microelectromechanical Systems, 17(2), 393-401.
[https://doi.org/10.1109/JMEMS.2008.918400]
-
Jo, B.-H., Van Lerberghe, L. M., Motsegood, K. M., Beebe, D. J., (2000), Three-dimensional micro-channel fabrication in polydimethylsiloxane (PDMS) elastomer, Journal of Microelectromechanical Systems, 9(1), 76-81.
[https://doi.org/10.1109/84.825780]
-
Hong, K. Y., Lee, D.-K., Kim, S., Cho, Y. H., (2019), Fabrication of anisotropically oleophobic surface with inverse-tapered structure using micromolding in capillaries and microtransfer molding, Journal of the Korean Society for Precision Engineering, 36(4), 413-418.
[https://doi.org/10.7736/KSPE.2019.36.4.413]
-
Yoon, R. R., Kim, S. M., Han, A., Cho, Y. H., (2017), Fabrication of pmma acoustophoretic microfluidic chip using plasma assisted bonding, Journal of the Korean Society for Precision Engineering, 34(5), 343-347.
[https://doi.org/10.7736/KSPE.2017.34.5.343]
Professor in the Department of Mechanical System Design Engineering, Seoul National University of Science & Technology. His research interest is electronic packaging and micro-LED.
E-mail: sdkim@seoultech.ac.kr
Graduated in the Department of Mechanical System Design Engineering, Seoul National University of Science & Technology. She is now working as a Semiconductor Process Development Engineer.
E-mail: jisujin96@naver.com
Assistant Research Scientist in Texas A&M University. Her research interest is lab-on-a-chip and organ-on-a-chip systems.
E-mail: songi86.han@gmail.com
Professor in the Department of Electrical & Computer Engineering, Texas A&M University. His research interest is microfluidics and lab-on-a-chip systems.
E-mail: arum.han@ece.tamu.edu
Professor in the Department of Mechanical System Design Engineering, Seoul National University of Science & Technology. His research interest is Bio-MEMS and Micro/Nano fabrication.
E-mail: yhcho@seoultech.ac.kr