
초음파 분사 노즐의 분사 범위에 따른 슬러리 막 두께의 변화가 재료제거율에 미치는 영향
Copyright © The Korean Society for Precision Engineering
This is an Open-Access article distributed under the terms of the Creative Commons Attribution Non-Commercial License (http://creativecommons.org/licenses/by-nc/3.0) which permits unrestricted non-commercial use, distribution, and reproduction in any medium, provided the original work is properly cited.
Abstract
Most of the consumables used in the CMP (Chemical Mechanical Planarization) process are discarded because it is difficult to reuse them. Slurry accounts for most of the consumables, so research is being conducted to reduce the amount of slurry used. A previous study explains that when the same amount of slurry is injected, the material removal rate is improved when the slurry is injected wide and thin instead of the tube nozzle, which is the conventional slurry injection method. However, there was no change in the injection method due to the problems of the injection method suggested in previous studies and the lack of follow-up studies. Thus, in this paper, an injection method through an ultrasonic spray nozzle is proposed to improve the problems of the injection method proposed in previous studies. Additionally, it is intended to calculate the slurry film thickness according to the spraying range and to explain the effect of the film thickness on the material removal rate.
Keywords:
Chemical mechanical planarization, Ultrasonic spray nozzle, Slurry film thickness, Material removal rate, Real wet area키워드:
화학적 기계적 평탄화, 초음파 분사 노즐, 슬러리 막 두께, 재료제거율, 실제 젖은 면적1. 서론
최근 반도체의 집적도 향상은 층 내에서는 공정 미세화를 통해 회로 선폭을 작게 만드는 방법과, 층 외에서는 다층 구조의 심화를 통해 이루어지고 있다. 이 두 가지 방법은 하나의 반도체 칩을 만드는데 들어가는 공정 수가 증가하는 결과를 야기했다. 증가한 공정 수만큼 공정들로 인한 불균일한 면을 평탄화하는 화학적 기계적 평탄화(Chemical Mechanical Planarization, CMP) 공정의 수도 증가했다[1]. CMP 공정 수의 증가는 공정에서 소모되는 소모재의 사용량 증가로 이어져, 소모재 시장이 커지는 주된 역할을 했다[2]. 소모재는 주로 패드, 컨디셔너, 슬러리로 분류되며 용량적으로 가장 큰 슬러리가 시장에서 가장 큰 비중을 슬러리가 차지하고 있다[3].
슬러리의 대부분은 재활용을 하지 못하고 폐기되며, 슬러리의 특성상 환경적 문제를 야기한다. 연구자들은 슬러리를 재활용하는 방법들과 슬러리의 특성을 강화하여 사용량을 줄이는 방법들을 연구해왔다[4,5]. 그러나 재활용의 경우 기존 방식의 공정 결과와 동일한 결과를 얻기 어려웠으며, 재활용 횟수가 증가할수록 공정 결과가 더 나빠지는 단점을 가진다. 슬러리 특성 강화의 경우, 강화를 위해 첨가된 화학물질 혹은 연마 입자가 다른 환경적 문제를 야기했다.
그래서 연구자들은 슬러리와 웨이퍼 간의 접촉에 주목하여 연구를 했다. Liao, et al., [6]은 다중의 튜브 노즐을 활용해 웨이퍼 전면에 슬러리가 균일하게 접촉하도록 하는 방안을 모색했으며, Lee [7]는 단일 분사 노즐을 활용해 슬러리를 주입하는 방법을 통해 공정 결과 향상을 모색했다. 이외의 비슷한 맥락의 연구들은 공통적으로 동일한 슬러리 주입량 기준, 기존 단일 튜브 노즐을 통한 주입 대비, 각 연구 방법을 통한 주입 시 재료제거율이 향상되는 결과를 얻었다고 말한다[8,9].
연구에서 제시한 방법들은 다르나 공통적으로 슬러리를 얇고 넓게 주입해야 한다고 말한다. 즉, 슬러리 주입 시 형성되는 슬러리 막 두께와 웨이퍼와 접촉하는 면적이 CMP 공정 결과에 영향을 준다는 걸 알 수 있다. 하지만 슬러리 막 두께를 균일 제어하는 것이 어렵기에 관련 연구가 미미하다. 본 연구에서는 슬러리 막 두께를 제어하기 위해 초음파 분사 노즐을 활용하고 분사 범위에 따른 막 두께의 차이가 CMP 공정에서 재료제거율에 어떤 영향을 미치는지에 대해 연구하였다.
2. 연구 배경
2.1 초음파 분사 노즐
단일 튜브 주입 방식과 스프레이 노즐 방식은 슬러리 주입 시 Figs. 1(a)와 1(b)와 같은 모습으로 주입된다. 튜브의 경우 좁고 두꺼운 형태로 슬러리가 주입되며, 이로 인해 사용되지 못하는 잔여 슬러리가 발생한다. 스프레이의 경우 얇고 넓은 형태로 주입되며, 튜브에 비해 잔여 슬러리가 적다. 그러나 액적 크기와 분사 범위 내 분사량이 균일하지 않아 불균일한 막 두께를 가진다. 균일한 분사가 기능하려면, 오리피스의 직경이 매우 작아야 하는데 이로 인해 입구가 막히는 현상과 비산현상 같은 문제가 발생한다[10].
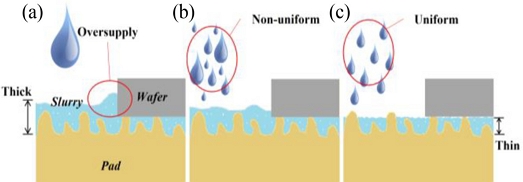
Schematic diagrams of slurry appearance according to injection method (a) Tube, (b) Spray nozzle, and (c) Ultrasonic spray nozzle
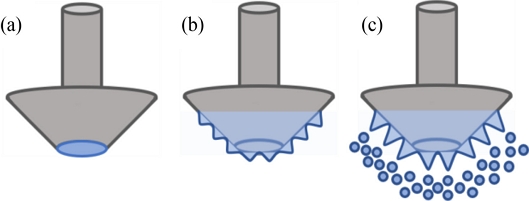
Mechanism of ultrasonic spray nozzle (a) Injection of liquid, (b) Vibration of nozzle, and (c) Droplet spraying by vibration exceeding the surface tension of liquid
이런 문제점 없이 균일 분사가 가능한 초음파 분사 노즐을 사용해 균일한 막 두께가 형성되게 하였고, 슬러리 주입량을 고정한 상태에서 분사 범위를 조절해 슬러리 막 두께를 변화시켜 실험을 했다. 단, 초음파 분사 노즐의 경우 노즐의 특성상 최대 사용 가능한 유량이 정해져 있다. 본 연구에서는 세라토크 사의 S40 모델을 최대 유량이 100ml/min로 주문 제작했다. S40의 경우 구동주파수 40 kHz이며, 일반 물 기준 액적 직경은 40 μm이다. 액적의 크기는 점성 외 다른 인자들도 복합적으로 영향을 주지만[11], 주로 점성에 따라 차이가 발생하며, 본 실험에서 사용하는 슬러리의 점성(25oC 기준 5 cP 이하)과 일반 물(25oC 기준 0.89 cP)의 점성의 차이는 액적의 크기에 영향 주지 않아 고려하지 않았다[12].
2.2 일반적인 웨이퍼와 접촉하는 슬러리 막 두께 계산
CMP 공정에서 필요한 슬러리 양을 계산하기 위해서는 고려되어야 할 사항들이 많이 있으나, 몇 가지 가정을 통해 간단히 표현할 수 있다.
(1) 웨이퍼와 패드의 움직임에 따른 슬러리의 유실은 무시한다.
(2) 패드의 그루브에 대한 영향은 무시한다.
위 가정에서 Wafer의 Oscillation 동작이 없다면, Fig. 4(a)와 같이 볼 수 있고, 슬러리 양은 접촉 면적의 중복과 무관하므로 CMP 공정시간(1분) 동안 패드와 접촉하는 웨이퍼의 총 면적은 식(1)과 같이 표현할 수 있다.
(1) |
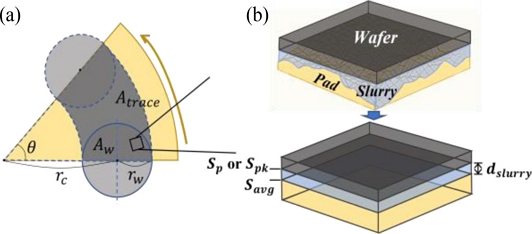
(a) Schematic diagram of wafer traces due to pad rotation, and (b) Slurry film thickness using geometric average
각도 θ는 패드의 분당 회전수(n)에 의해 결정되므로 식(2)가 되며, 총면적은 식(3)으로 표현된다.
(2) |
(3) |
식(3)에 대해 Figs. 4(b)와 같이 웨이퍼와 패드가 접촉하는 임의의 체적에서 슬러리가 차지하는 높이(최대 슬러리 막 두께) dslurry는 4(b)처럼 패드 돌기 최대 높이인 Sp와 표면의 중심 높이(높이 평균)인 Sava의 차를 이용해 식(4)로 나타낼 수 있다.
(4) |
식(4)는 패드 표면의 돌기의 변형이 없어야 성립이 되나 실제 패드는 웨이퍼 압력에 의해 패드 돌기의 변형이 발생한다. 패드 돌기 변형을 고려할 경우, Sp 대신 실제 접촉 높이에 가까운 줄어든 돌기 높이인 Spk를 사용해 계산하면 식(5)로 표현된다[13].
(5) |
Atotal에 dslurry를 곱해 CMP 공정에서 필요한 슬러리 양인 Qslurry를 계산할 수 있다.
(6) |
위 식(4)와 식(5)는 이론적으로 패드와 웨이퍼 사이에 가득찬 상황에 대해서만 가능하다. 가득 차지 않은 상황에서 슬러리 막 두께를 계산할 방법이 필요하다.
2.3 실제 젖은 면적 비율(Real Wet Area Ratio, RWA)을 이용한 막 두께 계산
Lu [14]는 웨이퍼 하부의 슬러리 막 두께를 측정하고자 웨이퍼 센터에 압력을 가하는 방식의 CMP 장비를 이용해 염색한 슬러리와 자외선을 통해 웨이퍼 상부에서 측정했다. 그러나 이와 같은 방법은 웨이퍼 전체 고른 압력을 가하는 현재 장비에는 사용할 수 없다. 또한, 주입되는 슬러리가 형성하는 막 두께는 패드 돌기에 의한 지형적인 특성도 고려해야 하므로 측정 방법에 한계가 있다. 동축 광원 현미경을 통해 패드를 관찰할 때, Figs. 5(b)와 5(d)와 같이 액체로 젖은 부분에서 빛의 반사 및 굴절에 의해 밝게 보이는 점을 이용해 실제 젖은 면적비(Real Wet Area Ratio, RWA)을 측정할 수 있다.
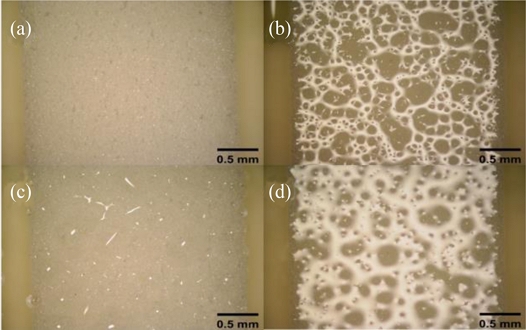
RWA on the pad using DIW (Deionized water) according to (a) Dry, (b) Conditioned, (c) Dry and spray injection, and (d) Conditioned and spray injection
단, Figs. 5(a)와 5(c)와 같이 건조 상태일 때는 볼 수 없으며, 액체의 응집에 의해 액체 표면에 곡률이 형성됨에 따라, 젖은 면적에 보정을 해야 하나 본 연구에서는 고려하지 않았다. 향후 보완이 필요하다. 또한 Figs. 6(a)처럼 액체가 완전히 패드를 덮어버리는 경우에는 6(b)처럼 RWA 측정이 불가하다. Fig. 7과 같이 동일 위치의 표면 형상 이미지와 RWA 이미지를 겹쳐보면, 원으로 표시한 부분의 돌출부(돌기)는 RWA 이미지에서 젖지 않는 모습을 볼 수 있다. 이러한 점을 이용해 다음과 같이 생각해 볼 수 있다.
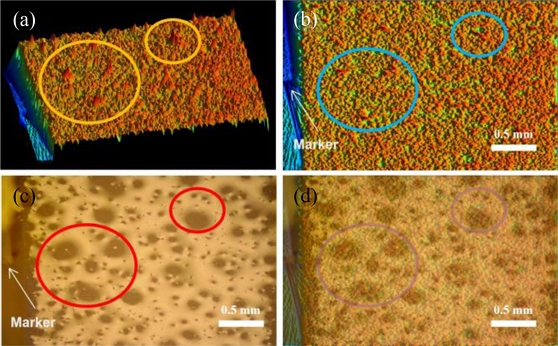
Comparison of surface shape of the sample and image of RWA: Confocal microscope image (a) 3D, (b) 2D, (c) RWA image, and (d) Overlap (b) and (c)
표면 형상에 대한 정보와 RWA (kRWA)를 안다면 Fig. 8과 같이 RWA (kRWA)는 표면 형상 정보를 이용해 계산되는 겉보기 면적비인 Bearing Area Ratio (kB)를 제외한 나머지이므로 식(7)과 같이 표현된다.
(7) |
식(7)을 통해 kB를 구하고, kB에 해당하는 특정 높이(h)를 알 수 있다. 즉, 수면의 높이를 구할 수 있다. Bearing Area Ratio를 이용해 h 이상의 높이에 대한 평균 높이(Sh)도 구할 수 있다.
(8) |
패드의 평균 높이(Sava)와 h 이상의 높이에 대한 평균 높이(Sh)는 기하학적 관점에서 Figs. 9(a)와 9(b)와 같이 볼 수 있다. 이를 이용해 슬러리 막 두께(dslurry)는 식(8)과 같이 표현되며, 표면의 형상 정보와 RWA (kRWA)를 통해 슬러리 막 두께를 계산할 수 있다.
3. 실험 방법 및 결과 분석
3.1 분사 범위에 따른 범위 내 RWA 측정 실험
RWA (kRWA)를 측정하기 위해서는 동축 광원 현미경의 측정 선반에 올려야 하므로 측정이 가능한 크기(30 × 30 mm)로 시편을 만들고 Fig. 10과 같이 실험 방법을 구상해 RWA를 측정했다. 실험 결과 Fig. 12와 같이 RWA 값은 94.5, 80.7, 68.6, 64.5%이다. 참조 데이터가 일정하지 않아 실험에 다소 영향을 주었을 것으로 판단되나, 전체적인 경향성에는 영향을 주지는 않았다. 실험 후, 시편을 건조하고 공초점 현미경(NS-3500)을 이용해 Fig. 13과 같이 표면의 형상 정보를 얻었다. 이를 활용해 Fig. 14와 같은 그래프를 얻었다. 위 데이터를 이용해 RWA에 따른 Sh를 계산하면 dslurry를 Fig. 15와 같은 그래프로 나타낼 수 있다.
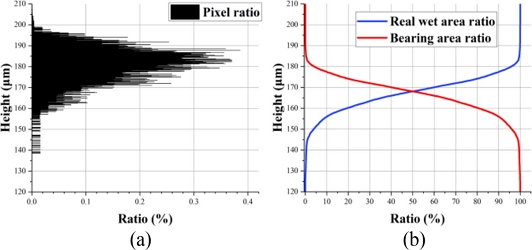
Graphs of (a) Ratio of the number of pixels by height to the total number of pixels, and (b) Ratio of bearing area and real wet area
Sh는 특성상 최소 Sava = 167.13 μm에서 최대 Sp = 207.81 μm 값을 가지며, 각 분사 범위에 따른 kRWA를 통한 Sh의 값은 175.56, 176.11, 178.07, 181.65 μm이며, 식(8)을 통한 dslurry는 8.43, 8.98, 10.94, 14.52 μm이 된다. 표면의 형상 정보를 바탕으로 Spk 값을 계산한 결과, Spk = 176.63 μm로 식(5)를 통한 dslurry = 9.5 μm이다. 실험 결과를 비교하면, 분사 범위 75 mm일 때의 dslurry 값이 Spk 값을 통한 dslurry 값에 가장 가깝다는 걸 통해 재료제거율이 가장 높게 나올 것으로 추측된다.
3.2 분사 범위에 따른 CMP 공정 실험
Table 1과 같이 CMP 공정 실험 조건을 설정하고 분사 범위를 변화시켜 3번 반복 실험을 진행했다. 실험 결과 Fig. 16과 같이 분사 범위에 따른 재료제거율 그래프를 얻었다. 실험 결과를 살펴보면 분사 범위 75 mm에서 가장 높은 재료제거율을 가지며, 이후 분사 범위가 줄어들수록 튜브 노즐을 이용한 실험 결과와 같아지는 경향을 보인다. 앞선 RWA 실험에서 Spk 값에 근접한 분사 범위 75 mm에서 가장 재료제거율이 높을 것이라 했던 추측한 것과 일치한다.
또한 Fig. 17의 그래프를 보면, 공정 시작 후 20초 동안 마찰력의 변화폭이 크며, 이후에는 변화폭이 감소하는 걸 알 수 있고, 분사 범위 75 mm에서 마찰력 변화폭 이 가장 작은 걸 알 수 있다. 이는 스틱-슬립 마찰 특성에 비춰 보면, 시간에 따라 패드의 잔류 슬러리 누적으로 인해 웨이퍼와 슬러리의 접촉 면적의 증가에 따른 영향으로 보이며, 마찰력 변화폭이 작을수록 실 접촉 면적이 넓다고 할 수 있다[15,16].
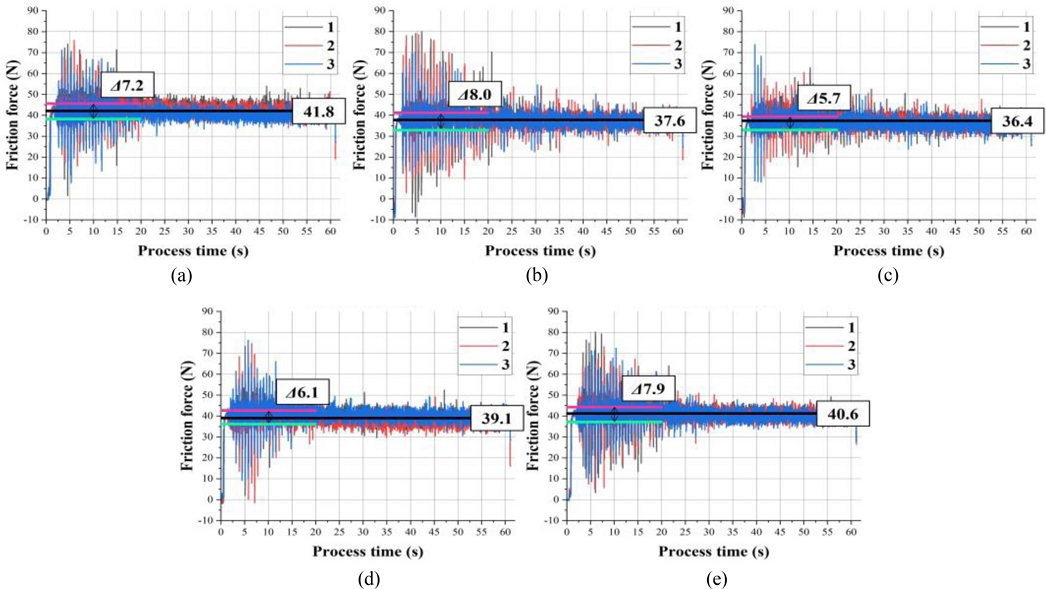
Graph of friction force, average of friction force for process and average of friction force for 20s according to spray range: (a) Tube, (b) 100, (c) 75, (d) 50, and (e) 25 mm
이러한 점들을 근거로 결과를 분석하면 Fig. 18과 같이 슬러리 주입량이 동일할 때, 좁은 분사 범위의 경우(Figs. 18(a)와 18(b)), 높은 슬러리 막 두께를 형성하지만 분사 범위가 슬러리와 웨이퍼의 접촉 면적을 저해하는 원인으로 작용하고 이로 인해 재료제거율이 감소한다. 반면, 넓은 분사 범위의 경우(Fig. 18(d)), 슬러리와 웨이퍼의 접촉 면적은 넓으나 슬러리 막 두께가 슬러리와 웨이퍼의 접촉을 저해하는 원인으로 작용한다. 따라서 슬러리 막 두께와 슬러리와 웨이퍼의 접촉 면적 두 가지를 동시에 만족시켜야 가장 높은 재료제거율을 얻을 수 있다고 판단된다.
4. 결론
본 연구에서는 스프레이 분사 주입의 단점을 보완하고자 초음파 분사 노즐을 이용하였다. 동일한 양의 슬러리를 주입할 때 분사 범위에 따라 패드 위 형성되는 슬러리 막 두께를 측정하고, 이에 따라 CMP 공정의 재료제거율이 어떻게 달라지는지, 그 이유는 무엇인지에 대해 웨이퍼와 슬러리의 접촉에 대한 관점에서 알아보았다.
실험 결과 분사 범위 75 mm일때, 슬러리 막 두께가 8.98 μm이고, 재료제거율은 3회 평균 2,579.4 Å/min으로 분사 범위를 제외한 동일 조건에서 2,460.8 Å/min인 튜브 노즐 대비 118.6 Å/min 만큼 더 많이 재료제거가 된다. 이러한 결과는 공정 압력과 패드 표면 형상 및 패드 재료의 물성에 의해 웨이퍼 하부로 들어 갈 수 있는 슬러리 막 두께의 한계가 정해지기 때문에 슬러리 주입량이 동일할 때, 슬러리 막 두께가 한곗값에 가까울수록 재료제거율이 높아지는 실험 결과를 보였다.
따라서 슬러리 주입량 대비 최대 재료제거율을 얻기 위해서는 웨이퍼와 패드 사이 공간을 다 채울 만큼의 슬러리 막 두께가 형성되야 한다고 판단된다. 판단의 근거로 분사 범위에 따른 슬러리 막 두께가 Spk를 이용해 계산된 슬러리 막 두께 값에 가까울수록 재료제거율이 높다는 점과 더불어 공정 중 마찰력의 변화를 근거로 들고, 그 이유를 설명했다. 향후 본 논문에서 제시하는 초음파 분사 노즐을 활용한 슬러리 주입 방식이 슬러리 막 두께를 제어하고, 이로 인한 슬러리 소비량을 감소에 도움이 되리라 예상한다.
NOMENCLATURE
Atotal : | Total Contact Area of the Wafer and Pad |
Aw : | Wafer Area |
Atrace : | Contact Area of the Sliding Wafer on the Pad |
rw : | Radius of Wafer |
rc : | Length between the Center of Pad and Center of Wafer |
θ : | Angle according to the Rotation of the Pad |
n : | Number of Revolutions |
Qslurry : | Total Amount of Slurry |
Sp : | Maximum Peak Height in Surface Area Profile |
Spk : | Reduced Peak Height in Surface Area Profile |
Savg : | Average of Heights in Surface Area Profile |
dslurry : | Slurry Film Thickness |
kB : | Ratio of the Bearing Area of a Surface to the Height |
kRWA : | Ratio of Real Wet Area |
h : | Specific Height Obtained through Bearing Area Ratio |
Sh : | Average of Heights above a Specific Height in Surface Area Profile |
Acknowledgments
This paper was presented at KSPE Spring Conference in 2021
이 연구는 Ebara Corporation의 지원을 받아 연구되었습니다(No. 201904190003).
REFERENCES
- Marty W. D., (2017), State of CMP materials, The Dow Chemical Company, 1-14. https://nccavs-usergroups.avs.org/wp-content/uploads/CMPUG2017/CMP-717-4-DeGroot-Dow.pdf
- CMC Critical Materials Council, CMP consumables US$2.9B in 2021 for IC Fabs. https://criticalmaterials.org/techcet-news/
- Davis, S., (2017), Techcet CMP consumables 2015 market update, Techcet, 1-31. https://nccavs-usergroups.avs.org/wp-content/uploads/CMPUG2015/CMP2015_7_5Davis.pdf
-
Lee, D., Lee, H., Jeong, H., (2016), Slurry components in metal chemical mechanical planarization (CMP) process: A review, International Journal of Precision Engineering and Manufacturing, 17(12), 1751-1762.
[https://doi.org/10.1007/s12541-016-0201-y]
-
Coetsier, C., Testa, F., Carretier, E., Ennahali, M., Laborie, B., Mouton-Arnaud, C., Fluchere, O., Moulin, P., (2011), Static dissolution rate of tungsten film versus chemical adjustments of a reused slurry for chemical mechanical polishing, Applied Surface Science, 257(14), 6163-6170.
[https://doi.org/10.1016/j.apsusc.2011.02.023]
-
Liao, X., Sampurno, Y., Zhuang, Y., Philipossian, A., (2012), Effect of slurry application/injection schemes on slurry availability during chemical mechanical planarization (CMP), Electrochemical and Solid-State Letters, 15(4), H118.
[https://doi.org/10.1149/2.009205esl]
-
Lee, D., Lee, H., Jeong, H., (2015), The effects of a spray slurry nozzle on copper CMP for reduction in slurry consumption, Journal of Mechanical Science and Technology, 29(12), 5057-5062.
[https://doi.org/10.1007/s12206-015-1101-2]
-
Meled, A., Zhuang, Y., Sampurno, Y. A., Theng, S., Jiao, Y., Borucki, L., Philipossian, A., (2011), Analysis of a novel slurry injection system in chemical mechanical planarization, Japanese Journal of Applied Physics, 50(5S1), 05EC01.
[https://doi.org/10.7567/JJAP.50.05EC01]
-
Philipossian, A., Borucki, L., Sampurno, Y., Zhuang, Y., (2015), Novel slurry injection system for improved slurry flow and reduced defects in CMP, Solid State Phenomena, 219, 143-147.
[https://doi.org/10.4028/www.scientific.net/SSP.219.143]
- Lee, D. S., (2020), Hybrid slurry supply system using ionization and atomization for sustainable CMP, Ph.D. Thesis, Pusan National University.
- Ju, E., La, W., Choi, W., (1999), A study on characteristics of the liquid atomization by ultrasonic, Transactions of the Korean Society of Automotive Engineers, 7(5), 31-39.
-
Mandato, S., Rondet, E., Delaplace, G., Barkouti, A., Galet, L., Accart, P., Ruiz, T., Cuq, B., (2012), Liquids’ atomization with two different nozzles: Modeling of the effects of some processing and formulation conditions by dimensional analysis, Powder Technology, 224, 323-330.
[https://doi.org/10.1016/j.powtec.2012.03.014]
- Jeong, H., Lee, H., Choi, S., Shin, W., Lee, H., Jeong, H., (2010), Simplification of microtopography on CMP pad using surface roughness parameters, Proceedings of the Korean Society of Precision Engineering Conference, 153-154.
-
Lu, J., Rogers, C., Manno, V., Philipossian, A., Anjur, S., Moinpour, M., (2004), Measurements of slurry film thickness and wafer drag during CMP, Journal of the Electrochemical Society, 151(4), G241.
[https://doi.org/10.1149/1.1649986]
-
Lee, H., Park, B., Seo, H., Park, K., Jeong, H., (2005), A study on the characteristics of stick-slip friction in CMP, Journal of the Korean Institute of Electrical and Electronic Material Engineers, 18(4), 313-320.
[https://doi.org/10.4313/JKEM.2005.18.4.313]
- Lee, H.-S., Park, B.-Y., Jeong, S.-H., Jeong, J.-W., Seo, H.-D., Jeong, H.-D., (2005), A study on stick-slip friction and scratch in Cu CMP, Proceedings of the Korean Institute of Electrical and Electronic Material Engineers Conference, 653-654.
He candidate the M.S. degree in the Department of Mechanical Engineering, Pusan National University. His research interest slurry supply system.
E-mail: snheo@pusan.ac.kr
Ph.D candidate in the Department of Mechanical Engineering, Pusan National University. His research interest is modeling of pattern CMP profile.
E-mail: shjeong@pusan.ac.kr
She candidate the M.S. degree in the Department of Mechanical Engineering, Pusan National University. Her research interest CMP contact mechanism.
E-mail: minji97@pusan.ac.kr
He candidate the M.S. degree in the Department of Mechanical Engineering, Pusan National University. His research interest machine.
E-mail: pyu0131@pusan.ac.kr
Professor in the Department of Mechanical Engineering, Pusan National University. His research fields include chemical mechanical polishing (CMP), grinding, polisher and consumable design, and post-CMP cleaning.
E-mail: hdjeong@pusan.ac.kr