
고온 하이브리드 클래딩 공정을 이용한 금속 적층품의 물성제어 특성 연구
Copyright © The Korean Society for Precision Engineering
This is an Open-Access article distributed under the terms of the Creative Commons Attribution Non-Commercial License (http://creativecommons.org/licenses/by-nc/3.0) which permits unrestricted non-commercial use, distribution, and reproduction in any medium, provided the original work is properly cited.
Abstract
A hybrid cladding technology was developed by combining direct energy deposition (DED) and ultrasonic nanocrystal surface modification (UNSM). This is an effective process to control the mechanical properties inside the metal-clad layer, but the scope to improve the internal properties is low. Therefore, in this study, the UNSM process was applied while heating at 300 and 600oC to increase the effectiveness of this hybrid additive process. To validate the characteristics of this method, a study on the cross-sectional properties upon application of heating was conducted. Hybrid cladding at 300 degrees produced improvements- over a 40% larger area than the results at room temperature. At 600 degrees, the hybrid cladding improved mechanical properties over a larger area by nearly 2 times. In this study, the characteristics of the room-temperature and the high-temperature hybrid cladding process were analyzed. The proposed method shows a high improvement effect and is a promising method to improve the internal mechanical properties of the cladded layer.
Keywords:
High temperature hybrid cladding, Cladding, Direct energy deposition, Ultrasonic nanocrystal surface modification, Internal mechanical properties키워드:
고온 하이브리드 클래딩, 클래딩, 직접 에너지 적층, 초음파 나노표면개질, 내부 기계적 특성1. 서론
금속 제품의 마모 특성의 개선은 냉간 및 열간 프레스 금형[1] 또는 가스 터빈[2] 및 연소 엔진[3]과 같은 에너지 장비를 사용하는 수많은 분야에서 주요 관심사이다. 고온 열화는 소재의 기계적 및 물리적 특성을 저하시켜 다양한 시스템 문제를 야기한다. 따라서 인코넬 합금 또는 세라믹 기반 소재로 제품을 만들거나 클래딩 방식을 사용하여 고온 마모 특성을 개선하기 위한 연구가 진행되고 있다[4]. 선행 연구에 따르면, 인코넬 소재는 다른 금속에 비해 내열성과 내마모성이 우수하여 고온 기계 부품에 많이 활용되고 있다. 최근에는 직접 에너지 적층(Direct Energy Deposition, DED) [4-6]과 같은 적층 기술을 활용하여 복잡한 형상을 가진 표면에도 클래딩 공정을 적용할 수 있게 되었다.
고온 마모 특성을 향상시키기 위해 하이브리드 클래딩 공법이 지속적으로 연구되고 있다. 레이저 기반 클래딩 및 표면 처리 공정과 결합된 하이브리드 클래딩 공정은 항공우주, 자동차, 조선, 중장비 등 다양한 산업 분야에서 더 높은 효율, 향상된 표면 품질, 비용 절감을 필요로 한다[7-9]. 또한 고출력 레이저와 같은 첨단 레이저 기술의 발전으로 다양한 응용 분야와 산업에서 레이저 기반 클래딩 및 표면 처리 공정을 사용할 수 있게 되었다[10-12]. 전반적으로 레이저 기반 클래딩 및 표면 처리 공정의 혼합 공정 시장의 추세는 더 높은 효율성, 향상된 표면 품질 및 비용 절감에 대한 요구로 인해 통합 및 다기능 표면을 증가시키는 방향으로 나아가고 있다.
이전 연구에서는 기계적 특성을 개선하기 위한 하이브리드 클래딩 공정의 후처리 기술로 가공[13-15], 재용융[16-18], 피닝[19-21], 초음파 나노결정 표면 개질(Ultrasonic Nanocrystal Surface Modification, UNSM) [22-28]을 포함한 물리적 표면 처리 작업이 활용되었다. 이 물리적 표면 처리 방법은 환경 친화적이며 다른 방법보다 빠릅니다. 하이브리드 클래딩 공정에 적용된 UNSM 공정에 대한 선행 연구에는 다음과 같은 후처리 공정이 포함된다[25]. Kim 등은 적층된 M4 소재에 UNSM 공정을 적용하여 표면 거칠기가 88.3%, 내마모성이 85.7% 개선되었다[22]. Yu 등은 회주철 소재를 DED 공정으로 복원한 후 UNSM 공정으로 표면 내마모성을 개선했다. 그 결과 마모율이 거의 0%에 가까웠다[23]. 그 외에도 다양한 피닝 공정과 혼합하여 기계적 특성 개선을 한 많은 연구가 있다. 그러나 대부분의 조사는 클래딩 층의 기계적 경도 또는 강도를 증가시켜 표면 마모를 방지하기 위해 UNSM 및 레이저 샷 피닝과 같은 표면 처리 기술을 포함했다[24-28].
따라서 우리는 고온 마모 특성을 극대화하기 위해 내부 및 외부 내마모성을 높이기 위해 UNSM과 클래딩 공정을 결합한 하이브리드 클래딩 공정이라는 새로운 적층 접근법을 제안한다. 이러한 기본 개념을 바탕으로 깊이에 상관없이 내부 기계적 물성을 향상시킬 수 있는 적층 방법을 개발하고자 했다. 특히 고온에서의 내마모성을 향상시키기 위한 하이브리드 클래딩 방식의 타당성을 조사했다.
2. 소재 및 실험방법
2.1 실험 소재 및 하이브리드 클래딩 장비
본 연구에서는 100 × 50 × 10 mm SUS316 기판을 사용했으며, 표면을 에탄올로 초음파 세척하여 남아있는 불순물을 제거했다. 클래딩 공정에 사용된 시중에서 판매되는 인코넬 718 분말 입자는 구형이며 크기는 50-150 μm이다. 파우더는 산화를 방지하기 위해 사용하지 않는 동안 진공 건조기에 보관했다.
DED 장비 기반 클래딩 모듈의 모식도를 Fig. 1에 나타내었다. Fig. 1(a)에 나타내었듯이 1 kW 디스크 레이저(RFL-C1000, Raycus, China) 를 사용하며 3축 CNC로 구성된다. 분말 공급가스로는 산화를 방지하기 위해 Ar 가스를 사용했다. 또한 제어시스템이 탑재된 PC, 레이저에서 발생하는 열을 줄이기 위한 냉각 시스템, 분말을 저장하는 분말 호퍼, 분말을 이송하는 분말공급기 등이 포함되어 있다. Figs. 1(b)는 1(a)의 a 부분을 확대하여 DED 공정의 메커니즘을 나타낸 모식도이다. 4개의 파우더 분사 장치를 통해 파우더가 사방으로 분사되고, 대물렌즈를 통해 파이버 레이저가 집속되어 조사된다. 그 다음 집중된 레이저에 의해 모재와 파우더가 녹아 상온에서 급속 냉각되어 평균 0.25mm의 클래딩 두께가 생성된다.
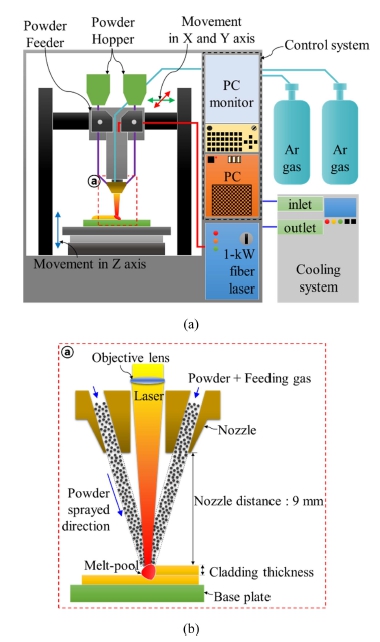
(a) Schematic diagram of laser cladding process, (b) a is schematic diagram of the cladding mechanism
초음파 나노 결정 표면 개질법(UNSM, Design Mecha, South Korea)의 개략도를 Fig. 2(a)에 나타냈다. UNSM 공정은 최대 20 kHz의 초음파 진동에 기반한 동적 하중과 무게 추를 이용한 정적 하중을 받는 금속 혼으로 대상 표면을 1,000-10,000회/mm2로 타격하여 국부적인 소성 및 탄성 변형을 일으킨다. 또한 결정립 미세화 과정에서 상당한 잔류 압축 응력이 발생한다. 이 처리 후에는 처리된 표면의 강도와 경도가 크게 증가한다. UNSM 공정은 초음파 발생기, 정하중을 가하는 무게 추, 진폭을 증폭하는 증폭기 등으로 구성되어 있다. 그리고 고온 하이브리드 클래딩 공정을 위해 가열 베드, 온도 컨트롤러, 열전대를 통합했다. 본 장비의 온도 컨트롤러를 통하여 열전대가 감지한 온도를 이용하여 가열 베드의 온도를 일정하게 유지할 수 있다. 그리고 Figs. 2(b)는 2(a)의 b 부분을 확대하여 UNSM 메커니즘을 나타낸 모식도이다. 초음파 진동 에너지로 증폭된 초음파를 손실 없이 전달하는 혼과 직경 2.4 mm의 텅스텐 카바이드 볼 팁이 타겟 표면을 타격한다. 타격을 입은 부분은 결정립 미세화를 나타내게 되어 강도 및 경도 향상, 압축잔류응력 발생 등의 효과를 확보할 수 있다.
2.2 실험 방법
하이브리드 클래딩 공정을 위한 클래딩 공정과 UNSM 공정의 공정조건을 선정했다. 클래딩 공정은 기본적으로 UNSM 처리 후 클래딩 공정을 적용하였을 때, 표면처리 효과가 남아있는 공정조건을 선정했다. 레이저 출력 350 W, 파우더 공급 속도 10 g/min, 가스 유량 3 L/min, 스캔 속도 800 mm/min으로 선정했다. 그리고 UNSM 공정의 공정 조건은 하중 15 kgf, 이송 속도 800 mm/min, 주파수 20 kHz, 간격 0.05 mm로 결정했다[29].
하이브리드 클래딩 공정 절차는 Fig. 3에 나타냈다. 이 공정은 기본적으로 DED 공정과 UNSM 공정의 반복으로 구성된다. 먼저Fig. 3의 1과 같이 DED 공정을 통해 1.0 mm 두께의 클래딩부를 형성했다. 이후 2와 같이 클래딩부의 표면에 클래딩 방향과 수직 방향으로 UNSM 표면 처리를 수행했다. 가열 베드의 적용에 따라 하이브리드 클래딩 공정을 적용했으며, 이 공정으로 약 1.0 mm의 두께로 만들었다. 그리고 가열 베드를 통하여 시험편을 가열한 온도는 300과 600oC의 온도를 적용했다. 그리고 3과 같이 UNSM 처리된 표면에 다시 한번 DED 공정을 이용한 클래딩부를 1.0 mm로 제작했다. 위의 2와 3을 반복하여 제조를 했으며, 이 반복과정을 통하여 하이브리드 클래딩부를 5.0 mm의 두께를 가질 때까지 반복하여 제작했다.
3. 결과 및 토의
3.1 경도 측정 결과
하이브리드 클래딩 공법 후 경도 시험 결과를 Fig. 4에 나타냈다. 경도 측정은 마이크로 경도 측정 장비(HM-112, Mituyoto, Japan)를 사용하여 하이브리드 클래딩 공정으로 제작된 시편의 경도를 0.1 kgf의 힘을 가하여 A-A' 단면에서 테스트했다. Fig. 4(a)는 상온에서 하이브리드 클래딩의 경도 테스트 결과를, Fig. 4(b)는 300ºC에서 가열한 하이브리드 클래딩의 경도 테스트 결과를 나타낸다. 그리고 Fig. 4(c)는 600ºC에서 가열한 하이브리드 클래딩의 경도 시험 결과를 나타냅니다.
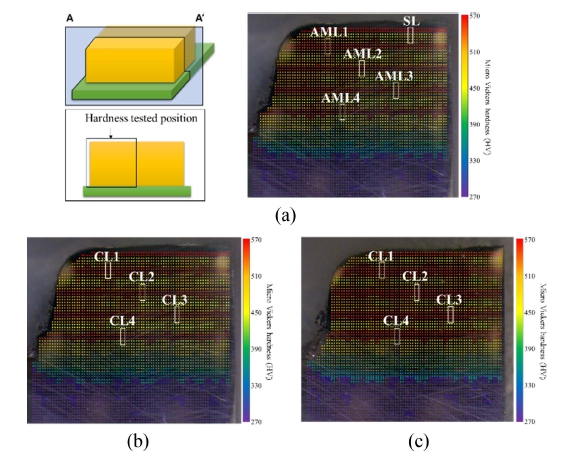
Mapping of hardness data for hybrid cladding process under (a) Room temperature (RT), (b) 300oC and (c) 600oC
Fig. 4(a)에 표시된 상온에서의 하이브리드 클래딩의 경우, 클래딩 된 전체 섹션의 평균 경도는 518 Hv인 반면, UNSM 처리된 층의 평균 경도는 565.75 Hv였다. 또한 Fig. 4(b)와 같이 300oC에서 가열한 하이브리드 클래딩 공정의 경우, 전체 하이브리드 클래딩 영역의 평균 경도는 568.0 Hv입니다. 600oC에서 가열된 하이브리드 클래딩의 경우, Fig. 4(c)와 같이 전체 하이브리드 클래딩 영역의 평균 경도는 523 Hv이고, UNSM 처리층의 평균 경도는 570.50 Hv이다.
일반적으로 제조품의 내부에서 경도의 급격한 변화가 있는 경우에는 응력집중이 발생할 수 있으며, 이는 클래딩 공정을 수행하는데 박리현상이나 내부 결함의 발생으로 연결될 수 있다. 본 연구에서는 이러한 결함의 발생을 줄일 수 있는 공정조건의 선정으로 문제 발생을 최소화했다.
이러한 경도 테스트 결과를 통해 몇 가지 중요한 점을 확인 할 수 있다. 첫째, 하이브리드 클래딩 공정이 수행된 두 공정 모두에서 UNSM 공정으로 인해 경도가 증가된 층이 생성된 것으로 확인되었다. 이는 상위 층의 클래딩 부분이 클래딩된 후에도 UNSM 공정의 결정립 미세화 부분이 지속된다는 것을 나타낸다. 또한, 이러한 이유로 내마모성 시험을 수행하면 UNSM으로 처리된 영역이 향상된 내마모성을 나타낼 것으로 예상된다. 또한 하이브리드 클래딩을 적용하면 클래딩만을 사용하여 클래딩한 시험편보다 경도가 더욱 우수하다. 이는 UNSM 공정이 적용된 층의 입자가 미세화되면서 경도와 내마모성이 향상되기 때문으로 판단된다. 마지막으로 가열한 하이브리드 클래딩은 다른 두 가지 방법보다 더 높은 경도를 나타냈다. 이러한 효과는 금속이 열을 받으면 부드러워지는 성질에 기인할 것으로 판단된다[30]. 따라서 가열 공정을 적용한 하이브리드 클래딩 공정이 내마모성을 가장 크게 향상시킬 수 있을 것으로 예상된다.
3.2 조직학적 분석
조직학적 분석을 위하여 후방산란전자 회절패턴 분석(Electron Backscatter Diffraction, EBSD)을 사용했으며, EBSD 측정을 위하여 측정을 위한 5 × 5 × 10 mm의 크기로 제작했으며, 측정 정밀도 확보를 위해 마운팅 및 폴리싱을 수행하여 매끄러운 표면으로 만들었다. 본 연구에서는 EBSD 측정을 위하여 실시간 전계 방출형 주사현미경 장비(JSM7200F, JEOL Ltd., Japan)를 사용하여 측정을 했다.
가장 먼저 상온에서의 내부 및 표면 처리가 다른 UNSM의 특성을 비교하기 위해 EBSD 이미지, 입자 크기 분포 및 경도 분포 그래프를 Fig. 5에 표시했다. Fig. 5(a)에서는 EBSD 측정 결과를 나타냈다. Fig. 4(a)에도 표시된 클래딩 후 가장 표면에 UNSM 처리가 된 표면층(Surface Layer, SL) 은 약 164.6 μm의 UTD가 관찰되었고, 하이브리드 클래딩 된 내부에 UNSM을 처리한 클래딩 층(Cladded Layer, CL) 의 경우 103.1 μm의 UTD 가 관찰되었다. Fig. 5(b)에 나타낸 입자 크기 분포를 나타냈다. 이 분포에서 10 μm 이하의 크기를 가지는 영역을 결정립 미세화 영역(Grain Refined Zone, GRZ)으로 확인되었으며, 결정립 크기가 10 μm 이상이면서 미세화 되지 않은 결정립보다 작은 크기를 가지는 영역을 결정립 미세화 유효 영역(Grain Refined Effected Zone, GREZ)으로 구분했다. 이를 통하여 CL과 SL의 각 영역의 깊이를 확인했으며, CL의 경우 GRZ의 깊이가 약 100 μm, GREZ의 깊이가 약 200 μm을 나타났다. 그리고 SL의 경우 GRZ의 깊이가 175 μm, GREZ의 깊이가 200 μm로 나타났다. 이 두 결과를 통하여 SL의 GRZ의 깊이가 CL보다 깊게 나타난다는 것을 알 수 있다. 마지막으로 Fig. 5(c)는 깊이방향의 경도 측정 결과를 나타낸 것으로, SL의 경우 약 175 μm 깊이에서 경도가 급격히 감소하는 것을 확인할 수 있다. 또한 최대 경도는 두 위치에서 유사한 값을 나타냈다. 이러한 결과를 통해 CL에서 UNSM 처리 후 결정립 미세화 된 표면 위에 클래딩 공정을 적용하면서 재용융으로 인해 열영향을 받아 결정립 미세화 깊이가 감소한 것으로 판단된다.
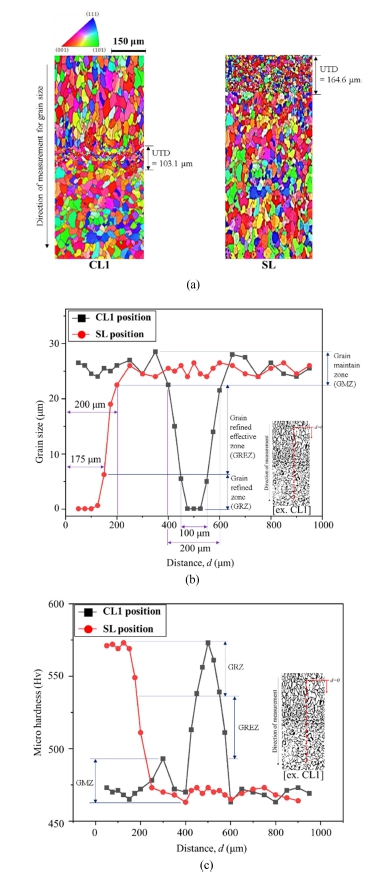
Comparison of (a) EBSD image; (b) distribution of grain size; and (c) distribution of hardness; of surface layer (SL) and inner cladded layer (CL) by hybrid cladding under RT
Figure 6에는 상온 하이브리드 클래딩에 따른 CL1부터 CL4까지의 EBSD 이미지, 결정립 크기 분포 및 경도 분포 결과를 나타냈다. Fig. 6(a)는 EBSD 이미지 측정 결과이며, CL의 평균 깊이는 약 98.5 μm로 나타났다. CL1은 103.1 μm, CL2는 103.7 μm, CL3은 98.5 μm, CL4는 88.5 μm의 UTD를 나타냈다. CL1에서 CL4로 깊어질수록 UTD가 감소하는 것을 볼 수 있는데, 하이브리드 클래딩을 적용할수록 지속적인 열로 인해 결정립 미세화 효과가 줄어들기 때문으로 볼 수 있다. Fig. 6(b)에서 볼 수 있듯이 모든 층(CL1-CL4) 의 GRZ 에서 약 100 μm의 깊이로 결정립 미세화가 관찰되었다. GREZ의 깊이 또한 약 200 μm로 확인되었다. Figs. 6(c)는 경도 분포를 나타낸 것으로, 6(b)와 유사한 결과를 보였다. 최대 경도는 565.75 Hv로 확인되었다.
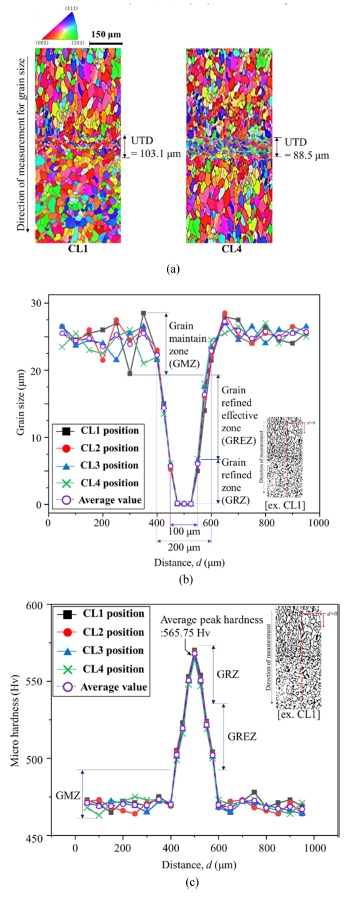
Comparison of (a) EBSD images, (b) grain size distribution, and (c) hardness distribution of cladded layer (CL1-CL4) by hybrid cladding under RT. The UNSM treated depth (UTD) of the cladded layers was found to be around 98.45 μm on average
Fig. 7에는 300oC에서 하이브리드 클래딩 공정에 대한 EBSD 측정 결과, 결정립 크기 분포 및 경도 분포가 나타냈다. Fig. 7(a)에서는 EBSD 측정 결과를 기반으로 각 층의 UTD 값을 비교했다. CL1은 156.2 μm, CL2는 142.3 μm, CL3은 135.4 μm, CL4는 128.5 μm의 UTD를 나타내었으며 평균 결정립 크기는 약 140 μm였다. 이 결과는 모재와 가까운 위치일수록 UTD가 작아진다는 것을 확인할 수 있었다. 그리고 Fig. 7(b)에서 볼 수 있듯이, 모든 위치에서 GRZ는 약 150 μm로 결정립 미세화가 관찰되었으며, GREZ의 깊이는 약 200 μm로 확인되었다. 또한 Fig. 7(c)에 경도 분포를 나타내었는데, 가장 평균 결정립 크기가 가장 작은 위치에서 568.0 Hv의 최대 경도를 나타냈다.
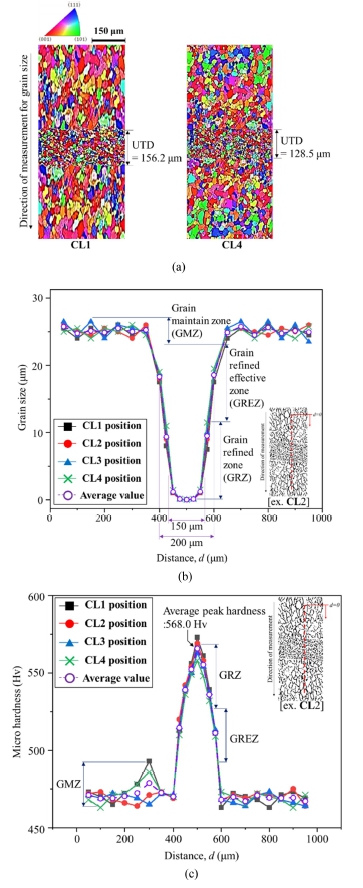
Analysis of (a) EBSD images, (b) grain size distribution, and (c) hardness distribution of cladded layer (CL1-CL4) by hybrid cladding under 300ºC. The UTD of the cladded layers was found to be approximately 140 μm on average
마지막으로 Fig. 8은 600oC에서 하이브리드 클래딩 공정에 대한 EBSD 측정 결과, 결정립 크기 분포, 경도 분포를 나타낸 것이다. Fig. 8(a)은 EBSD 측정 결과를 나타내었으며, 각 층의 UTD를 비교했다. CL1부터 CL4까지의 UTD는 각각 203.8 μm, 187.7 μm, 176.2 μm, 그리고 160.0 μm로 나타났으며, 전체 평균 결정립 크기는 약 180 μm였다. 그리고 Fig. 8(b)는 결정립 크기 분포를 나타낸 것으로, 모든 CL의 위치에서 GRZ는 약 200 μm의 결정립 미세화가 관찰되었으며, GREZ의 깊이는 250 μm로 확인되었다. 또한, Fig. 8(c)는 경도 분포를 나타내었으며, 가장 결정립 미세화 효과가 큰 위치에서 570.5 Hv의 최대 경도를 나타냈다.
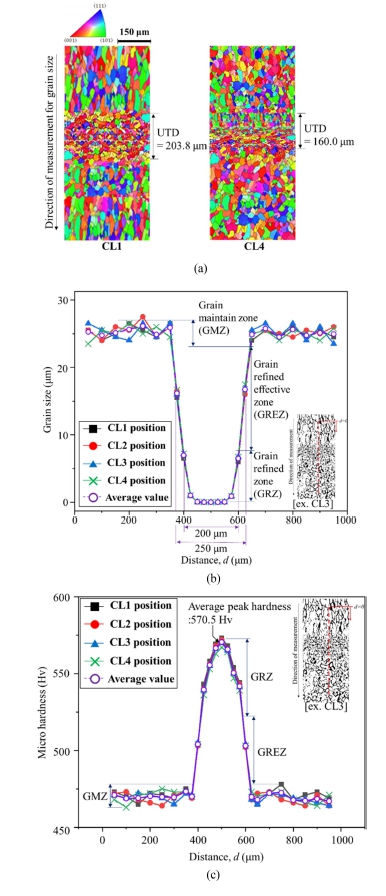
Analysis of (a) EBSD images, (b) grain size distribution, and (c) hardness distribution of cladded layer (CL1-CL4) by hybrid cladding at 600ºC. The UTD of the cladded layers was found to be about 182 μm on average
또한, Fig. 8(c)는 경도 분포를 나타냈으며, 가장 결정립 미세화 효과가 큰 위치에서 570.5 Hv의 최대 경도를 나타냈다. 각 결과에서 UTD는 CL1에서 CL4로 감소하는데, 이는 열누적에 의한 것으로 판단된다. CL4가 가장 깊은 위치에 있고 기본 재료에 가장 가깝기 때문에 그 위에 클래딩 공정을 적용하면서 열을 지속적으로 받기 때문이다. 이 결과를 통하여 고온 UNSM 공정의 적용을 통하여 더 깊은 효과를 확보할 수 있기 때문에 하이브리드 클래딩 공정에서 고온 UNSM 공정을 통해 내마모성의 높은 개선 효과를 확보할 수 있을 것으로 판단된다.
공정에 따른 결정립 크기 분포와 경도 분포를 비교한 그래프는 Fig. 9에 나와 있다. Fig. 9(a)에는 결정립 크기를 나타내었으며, 300oC의 온도에서는 입자 미세화 효과가 크지 않았으나, 600oC의 온도에서 하이브리드 클래딩을 수행하면 그 효과가 급격히 증가하는 것을 볼 수 있다. 또한 공정에 따른 경도의 변화는 Fig. 9(b)에 나타냈다. 그리고 GRZ의 경도 분포 차이는 600ºC에서 다른 두 공정보다 훨씬 더 넓게 분포되어 있다. 이러한 결과를 통해 하이브리드 클래딩 공정은 600ºC의 온도에서 효과적인 공정이라는 결론을 내릴 수 있다.
4. 결론
금속 제품의 내마모성을 극대화하기 위해 금속 클래딩과 UNSM 처리를 반복하여 내부 기계적 특성을 향상시킬 수 있는 하이브리드 클래딩 공정을 설명한다. 300oC와 600oC에서 하이브리드 클래딩 공정의 효과를 평가하기 위해 EBSD 이미지를 사용하여 미세 구조 변화를 연구하고 단면의 경도를 측정했다. 그 결과 상온과 300oC에서 하이브리드 클래딩 공정의 적용은 미약한 개선이 나타났고, 600oC에서 하이브리드 클래딩 공정에서 급격한 개선이 관찰했다.
또한 하이브리드 클래딩 공정을 사용하여 결정립 미세화가 발생한 깊이를 측정하였을 때, 그 깊이는 상온에서 표면처리를 적용한 경우보다 600oC에서 수행한 경우에 약 2배 가까이 나타났다. 이는 내마모성의 향상에 직접적인 영향을 미칠 수 있다는 것을 의미한다. 또한 이러한 결과는 고온 환경에서 UNSM 처리를 하면서 연해진 금속표면에 타격을 주어 그 효과가 더 크게 나타난 것으로 판단된다. 하이브리드 클래딩 공정의 적용과 기초 연구를 통해 UNSM 표면 처리 층의 존재를 입증했으며, 이를 통하여 기계적 특성의 향상에 큰 도움이 될 것이라고 판단했다.
Acknowledgments
This work was supported by the National Research Foundation of Korea (NRF) grant funded by the Korea government (No. 2019R1A5A808320112) and Korea Basic Science Institute (National Research Facilities and Equipment Center) grant funded by the Ministry of Education (No. 2021R1A6C101A449).
REFERENCES
-
Stott, F., (2002), High-temperature sliding wear of metals, Tribology International, 35(8), 489-495.
[https://doi.org/10.1016/S0301-679X(02)00041-5]
-
Pauzi, A. A., Ghazali, M. J., W. Zamri, W. F. H., Rajabi, A., (2020), Wear characteristics of superalloy and hardface coatings in gas turbine applications–a review, Metals, 10(9), 1171.
[https://doi.org/10.3390/met10091171]
-
Dudás, A., Laki, G., Nagy, A. L., Zsoldos, I., Hanula, B., Bartel, D., (2022), Wear behaviour of ceramic particle reinforced atmospheric plasma spray coatings on the cylinder running surface of internal combustion engines, Wear, 502, 204373.
[https://doi.org/10.1016/j.wear.2022.204373]
-
Evangeline, A., Sathiya, P., (2019), Structure–property relationships of Inconel 625 cladding on AISI 316L substrate produced by hot wire (HW) TIG metal deposition technique, Materials Research Express, 6(10), 106539.
[https://doi.org/10.1088/2053-1591/ab350f]
-
Torres, H., Rojacz, H., Čoga, L., Kalin, M., Ripoll, M. R., (2020), Local mechanical and frictional properties of Ag/MoS2-doped self-lubricating Ni-based laser claddings and resulting high temperature vacuum performance, Materials & Design, 186, 108296.
[https://doi.org/10.1016/j.matdes.2019.108296]
-
Zhang, T., Jiang, F., Huang, H., Lu, J., Wu, Y., Jiang, Z., Xu, X., (2021), Towards understanding the brittle–ductile transition in the extreme manufacturing, International Journal of Extreme Manufacturing, 3(2), 022001.
[https://doi.org/10.1088/2631-7990/abdfd7]
-
Liu, H., Liu, J., Chen, P., Yang, H., (2019), Microstructure and high temperature wear behaviour of in-situ TiC reinforced AlCoCrFeNi-based high-entropy alloy composite coatings fabricated by laser cladding, Optics & Laser Technology, 118, 140-150.
[https://doi.org/10.1016/j.optlastec.2019.05.006]
-
Pole, M., Sadeghilaridjani, M., Shittu, J., Ayyagari, A., Mukherjee, S., (2020), High temperature wear behavior of refractory high entropy alloys based on 4-5-6 elemental palette, Journal of Alloys and Compounds, 843, 156004.
[https://doi.org/10.1016/j.jallcom.2020.156004]
-
Wang, G., Zhang, J., Shu, R., Yang, S., (2019), High temperature wear resistance and thermal fatigue behavior of Stellite-6/WC coatings produced by laser cladding with Co-coated WC powder, International Journal of Refractory Metals and Hard Materials, 81, 63-70.
[https://doi.org/10.1016/j.ijrmhm.2019.02.024]
-
Teixeira, M. F., Pacheco, J. T., da Silva, L. J., Rabelo, A., Pereira, M., Niño, C. E., (2021), Wear resistance of a Metco 1030A hard coating deposited on Hadfield steel by laser cladding for ore comminution application, The International Journal of Advanced Manufacturing Technology, 112, 1873-1884.
[https://doi.org/10.1007/s00170-020-06415-2]
-
Wei, C., Zhang, Z., Cheng, D., Sun, Z., Zhu, M., Li, L., (2020), An overview of laser-based multiple metallic material additive manufacturing: From macro-to micro-scales, International Journal of Extreme Manufacturing, 3(1), 012003.
[https://doi.org/10.1088/2631-7990/abce04]
-
Wang, R., Xu, S., Yue, Y., Wang, X., (2020), Thermal behavior of materials in laser-assisted extreme manufacturing: Raman-based novel characterization, International Journal of Extreme Manufacturing, 2(3), 032004.
[https://doi.org/10.1088/2631-7990/aba17c]
-
Wang, J., Liu, S., Xu, B., Zhang, J., Sun, M., Li, D., (2021), Research progress on preparation technology of oxide dispersion strengthened steel for nuclear energy, International Journal of Extreme Manufacturing, 3(3), 032001.
[https://doi.org/10.1088/2631-7990/abff1a]
-
Kranz, J., Herzog, D., Emmelmann, C., (2015), Design guidelines for laser additive manufacturing of lightweight structures in TiAl6V4, Journal of Laser Applications, 27(S1), S14001.
[https://doi.org/10.2351/1.4885235]
-
Careri, F., Imbrogno, S., Umbrello, D., Attallah, M. M., Outeiro, J., Batista, A. C., (2021), Machining and heat treatment as post-processing strategies for Ni-superalloys structures fabricated using direct energy deposition, Journal of Manufacturing Processes, 61, 236-244.
[https://doi.org/10.1016/j.jmapro.2020.11.024]
-
Kapil, S., Legesse, F., Negi, S., Karunakaran, K., Bag, S., (2020), Hybrid layered manufacturing of a bimetallic injection mold of P20 tool steel and mild steel with conformal cooling channels, Progress in Additive Manufacturing, 5, 183-198.
[https://doi.org/10.1007/s40964-020-00129-3]
-
dos Santos Paes, L. E., Pereira, M., Xavier, F. A., Weingaertner, W. L., Vilarinho, L. O., (2022), Lack of fusion mitigation in directed energy deposition with laser (DED-L) additive manufacturing through laser remelting, Journal of Manufacturing Processes, 73, 67-77.
[https://doi.org/10.1016/j.jmapro.2021.10.052]
-
Cho, S. Y., Shin, G. Y., Shim, D. S., (2021), Effect of laser remelting on the surface characteristics of 316L stainless steel fabricated via directed energy deposition, Journal of Materials Research and Technology, 15, 5814-5832.
[https://doi.org/10.1016/j.jmrt.2021.11.054]
-
Meng, L., Zeng, X., Hou, K., Hu, Q., Wang, D., (2019), Effect of laser cladding and laser-induction hybrid cladding coatings on the bending properties and fracture behavior of rails, Surface and Coatings Technology, 374, 1038-1050.
[https://doi.org/10.1016/j.surfcoat.2019.06.051]
-
Lu, H., Xue, K., Xu, X., Luo, K., Xing, F., Yao, J., Lu, J., (2021), Effects of laser shock peening on microstructural evolution and wear property of laser hybrid remanufactured Ni25/Fe104 coating on H13 tool steel, Journal of Materials Processing Technology, 291, 117016.
[https://doi.org/10.1016/j.jmatprotec.2020.117016]
-
Wang, Y., Shi, J., (2019), Microstructure and properties of Inconel 718 fabricated by directed energy deposition with in-situ ultrasonic impact peening, Metallurgical and Materials Transactions B, 50, 2815-2827.
[https://doi.org/10.1007/s11663-019-01672-3]
-
Zhang, X., Li, D., Geng, J., (2021), An approach to reduce stress and defects: a hybrid process of laser cladding deposition and shot peening, Rapid Prototyping Journal, 27(9), 1609-1616.
[https://doi.org/10.1108/RPJ-06-2020-0129]
-
Yu, J.-H., Lee, K. Y., Shim, D.-S., Park, S.-H., (2019), Metal embedding and ultrasonic nanocrystal surface modification technology for super wear-resistant mechanical parts, The International Journal of Advanced Manufacturing Technology, 101, 951-962.
[https://doi.org/10.1007/s00170-018-2920-y]
-
Kim, M.-S., Jo, Y.-K., Park, S.-H., Shim, D.-S., (2019), Application of ultrasonic nanocrystal surface modification for improving surface profile of DEDed AISI 316L, Journal of Mechanical Science and Technology, 33, 5659-5667.
[https://doi.org/10.1007/s12206-019-1108-1]
-
Kim, C., Park, S., Pyoun, Y., Shim, D., (2021), Effects of ultrasonic nanocrystal surface modification on mechanical properties of AISI D2 steel, International Journal of Precision Engineering and Manufacturing, 22(7), 1271-1284.
[https://doi.org/10.1007/s12541-021-00536-8]
-
Jo, Y.-K., Gil, Y.-W., Shim, D.-S., Pyun, Y.-S., Park, S.-H., (2021), Control of Local Hardness Gradient of Metal Surface by Inclined Surface Treatment Using Ultrasonic Nanocrystal Surface Modification, International Journal of Precision Engineering and Manufacturing-Green Technology, 8, 533-546.
[https://doi.org/10.1007/s40684-020-00303-6]
-
Chang, S., Pyun, Y.-S., Amanov, A., (2015), Wear and chattering characteristics of rail materials by ultrasonic nanocrystal surface modification, International Journal of Precision Engineering and Manufacturing, 16, 2403-2410.
[https://doi.org/10.1007/s12541-015-0310-z]
-
Yeo, I., Bae, S., Amanov, A., Jeong, S., (2021), Effect of laser shock peening on properties of heat-treated Ti–6Al–4V manufactured by laser powder bed fusion, International Journal of Precision Engineering and Manufacturing-Green Technology, 8, 1137-1150.
[https://doi.org/10.1007/s40684-020-00234-2]
-
Jo, Y.-K., Song, D.-B., Choi, J.-S., Suh, J., Kahhal, P., Park, S.-H., (2023), Higher wear-resistant surfacing at high temperatures using a hybrid cladding process, Materials & Design, 225, 111553.
[https://doi.org/10.1016/j.matdes.2022.111553]
-
Sui, S., Li, Z., Zhong, C., Zhang, Q., Gasser, A., Chen, J., Chew, Y., Bi, G., (2021), Laves phase tuning for enhancing high temperature mechanical property improvement in laser directed energy deposited Inconel 718, Composites Part B: Engineering, 215, 108819.
[https://doi.org/10.1016/j.compositesb.2021.108819]
He is a Ph.D. candidate in the School of Mechanical Engineering at Pusan National University. His research topics are development of cladding process and its application to surface mechanical characteristics.
E-mail: a01056057898@pusan.ac.kr
He is a Professor in the School of Mechanical Engineering at Pusan National University. His research fields are additive manufacturing, sheet metal forming, and nanofabrication.
E-mail: sanghu@pusan.ac.kr