
항공우주 응용을 위한 반사형 광학 시스템
Copyright © The Korean Society for Precision Engineering
This is an Open-Access article distributed under the terms of the Creative Commons Attribution Non-Commercial License (http://creativecommons.org/licenses/by-nc/3.0) which permits unrestricted non-commercial use, distribution, and reproduction in any medium, provided the original work is properly cited.
Abstract
The utilization of aerospace technology is growing to meet various requirements of climate change sensing, communications, and the military. Large aperture and precise reflective optical systems such as mirrors are needed to acquire high-quality data, and the requirements of lightness, low cost, and low deformation should also be met to operate in the space environment. In this review, we highlight the recent progress of reflective optical systems for aerospace applications. In particular, optical systems for artificial satellites and homing optics are mainly introduced, and optical and mechanical simulations are discussed according to operating environments. We also discuss various reflective optic designs, materials, and operating principles for aerospace applications, such as a homing head and optical payload. We hope that this review provides approaches for developing surveillance systems, exploring space, and addressing the climate crisis.
Keywords:
Reflective optical systems, Aerospace, Optical payload, Environment simulation, Optical mirror키워드:
반사형 광학 시스템, 항공우주, 광학 페이로드, 환경 시뮬레이션, 광학 거울1. 서론
우주 광학 및 항공우주 기술의 급속한 성장과 원거리 고해상도 관측 시스템에 대한 기술 요구가 증가됨에 따라 우주 광학계에 대한 중요성이 지속적으로 증가하고 있다. 망원경과 같은 이미징 시스템을 통하여 원거리 고해상도 이미지를 획득하기 위해서는 집광 능력이 큰 조리개를 갖는 광학계가 필요하며, 수차를 최소화하고 무게를 감소시키기 위하여 렌즈 대신 거울(Mirror)과 같은 반사형 광학계가 주로 사용된다[1,2]. 위성용 우주 광학계의 경우 발사체를 이용하여 광학계를 우주 환경에 이동시켜야 함으로 조리개 구경의 증가는 발사 비용을 증가시키는 문제가 있다. 이러한 문제를 해결하고자 다양한 우주 광학계들은 경량화 설계가 고려되고 있으며, 우주 및 발사 환경에서 발생하는 진동 및 열 등의 외부 환경시뮬레이션을 통하여 미리 변형을 예측함으로 최적의 구조를 설계한다[3-5].
반사형 광학계 설계에 있어 재료 선택은 비용, 성능 및 신뢰성 측면에서 매우 중요하며 운용하고자 하는 환경에 맞게 적절한 소재를 선택하는 것이 필요하다[6]. 관측하고자 하는 거리, 시야각, 파장 및 탑재체의 크기에 따라서 광학설계가 달라지게 된다. 대부분의 위성 광학계는 주경과 부경을 포함하는 카세그레인(Cassegrain)식 구조를 채택하며, 이미지 해상도를 높이기 위하여 주경과 부경에서 반사된 빔을 최적 광학 구조를 통하여 집속한다[7,8]. 거울을 포함한 광학 부품들의 정렬, 편심 오차 등에 의하여 파면(Wavefront), 빔 직경 등의 광학적 특성이 변경 되므로 제작 및 운용 환경에 따른 광학계 오차를 분석하는 것이 매우 중요하다[9].
항공 응용분야에서는 빛을 추적하는 호밍(Homing) 시스템에도 반사형 광학시스템이 주로 사용된다. 호밍 광학계는 열을 통하여 방사되는 적외선을 감지함으로 실시간으로 대상 물체를 추적하는 역할을 수행한다. 호밍 광학계는 위성용 광학계와 달리 주로 대기 환경에도 사용되기 때문에 공기역학적 시뮬레이션에 대하여 환경 평가를 고려해야 하며, 레티클(Reticle)을 통하여 위치를 실시간으로 보정한다[10,11]. 운용 환경에 따라서 공기역학적 힘에 의한 광학계의 변형을 막기 위하여 돔 형태의 보호막을 장착하기도 한다. 또한, 움직이는 물체를 정확하게 추적을 하기 위해서는 예측 제어 알고리즘이 필요하며, 기존 좌표를 기반으로 벡터를 추정할 수 있다[12-14].
본 논문에서는 항공우주 응용을 위한 반사형 광학 시스템 연구 동향에 대하여 소개한다(Fig. 1). 지구 또는 우주 관측용 위성 및 호밍 시스템에 장착되는 광학 시스템에 대하여 주로 다루며 광학 설계 및 신뢰성 시뮬레이션에 대한 연구 소개도 포함한다. 가장 먼저 항공우주용 광학계의 핵심 부품인 반사형 광학 부품의 소재, 제작 공정 및 측정 방법에 대하여 연구 동향을 소개하고, 위성용 광학계 및 호밍 유도 시스템에 적용되는 반사형 광학 시스템에 대하여 구체적으로 설명한다. 각 절에서는 요구조건 및 최신 연구동향에 대하여 제시하며 각 시스템의 원리 및 최적화 방법에 대하여 소개한다.
2. 반사형 광학 부품
2.1 반사형 광학 부품 소재
반사형 거울의 소재로 세라믹, 유리 및 금속이 주로 사용된다(Table 1). 그 중에서도 우주형 광학계로 탄화규소(Silicon Carbide, SiC), 제로듀어(Zerodur), 용융 실리카(Fused Silica), 알류미늄 합금(Aluminum Alloy), 베릴륨(Beryllium, Be) 재료가 가장 많이 사용되며, 각각의 재료들은 비용, 열 안정성(Thermal Stability) 및 강성의 변수들에서 장단점이 있다[6]. 알루미늄 합금과 금속 소재는 열 안정성이 다른 소재 대비 낮은 단점이 있지만, 제조 및 처리가 쉽고 매우 가벼우며 비용이 저렴하다는 장점이 있다[15,16]. 또한 다이아몬드 정밀 선삭 가공(Singlepoint Diamond Turning, SPDT)와 같은 정밀 가공 기술을 이용하면 금속 거울의 높은 형상 및 광학적 정확도를 구현할 수 있다[17-19]. 이러한 알루미늄 합금의 장점을 이용하여 광역적외선 탐사 위성(Wide-field Infrared Survey Explorer)의 광학계는 금(Gold)이 코팅된 알루미늄 소재로 채택 되었다[20]. 금속 소재 중 베릴륨은 무게 대비 강성이 높고 열 안정성이 알루미늄보다 높아 제임스 웹 우주망원경(James Webb Space Telescope)의 주거울의 재료로 사용되었다[21]. 용융 실리카와 같은 유리 소재는 상용 렌즈로 많이 사용되나 높은 무게로 인하여 대형 우주 광학계 부품으로 이상적인 물질이 아니다. 하지만, 초소형 위성용 광학계의 경우 소구경의 광학계가 장착되기 때문에 거울 또는 렌즈 소재로 융용 실리카가 사용된다. 대표적인 예로 과학기술위성 3호(STSAT3)에 사용되는 광학계는 대부분 융용 실리카를 채택하였다[22]. 탄화규소재료는 높은 열 안정성 및 우수한 기계적 특성으로 인하여 우주 광학계에서 매우 잠재력이 높은 후보 물질 중 하나이다. 탄화규소와 같은 세라믹 기판 제작방법은 반응결합(Reaction Bonded), 열압착, 소결(Sintering) 및 화학 기상증착(Chaemical Vapor Deposition, CVD)이 있으며, 제작방법에 따라서 각 재료의 특성이 달라질 수 있다. 탄화규소는 다공성 및 미세구조 결합을 가지고 있어 가공이 어려우며, 표면 손상을 일으키지 않고 연삭 및 연마 작업을 수행하는 고정밀 가공 기술이 필요하다[23].
2.2 반사형 광학 부품 제작 방법
반사형 광학부품을 제작하는 방법은 크게 연삭 및 연마와 같은 기계적 가공과 3D 프린팅과 같은 적측형 제조(Additive Manufacturing)로 구분할 수 있다[24]. 기계적 가공의 경우 다양한 재료를 사용할 수 있으며 높은 형상정밀도를 구현할 수 있다는 장점이 있으나, 비구면 형상과 같은 표면 가공 시 가공의 복잡도가 높아진다. 반면, 적측형 제조는 재료 선택의 다양성이 제한되지만 자유 곡면을 제작하는데 용이하다.
기계적 가공을 통하여 화학기상증착 탄화규소(CVD SiC) 거울을 제작하기 위해서는 Fig. 2에 묘사된 공정 절차가 필요하다[25]. 탄화규소 소재를 합성하기 위하여 탄화규소 파우더와 용제를 혼합하여 젤 형태의 슬러리를 만들고, 젤을 주조하여 탄화규소 부품을 제작한다. 합성된 탄화규소 부품의 외형을 가공하여 곡률 형상을 제작하고 뒷면을 가공하여 경량화 작업을 진행한다. 화학기상증착 방법을 통하여 곡률 면에 탄화규소를 약 500 μm 증착시킨 후, 연삭과 연마를 통하여 곡률을 재가공한다. 화학기상증착 방법으로 증착된 표면이 고르지 못하기 때문에 추가적인 재가공이 필요하다. 광학적으로 반사도를 향상시키기 위하여 크롬(Cr), 알류미늄(Al), 이산화규소(SiO2), 오산화나이오븀(Nb2O5) 등의 재료를 적층하여 최종적으로 거울을 제작한다.
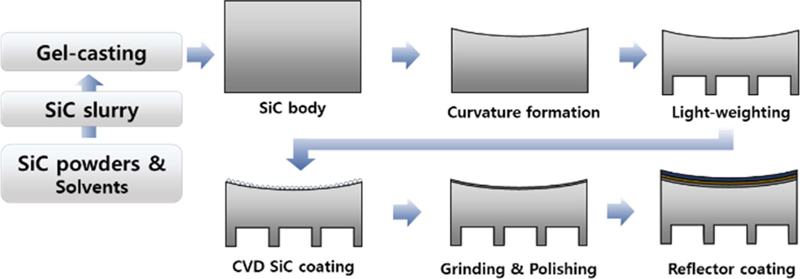
Schematic illustrations of CVD SSiC mirror manufacturing. After synthesizing the SiC body, the CVD SiC mirror is fabricated through mechanical processing, deposition, grinding, polishing, and reflective material coating
기계적 가공 방법을 통해 비구면 거울을 제작하기 위해서는 고정밀 가공 기술이 요구된다. 최근 헤테로세칼 스트레스 랩핑(Heterocercal Stressed Lapping) 연삭/연마, 컴퓨터-제어 광학표면(Computer-controlled Optical Surfacing, CCOS) 및 자기유동유체(Magnetorheological Finishing, MRF) 연마 기술을 통하여 직경 4.03 m의 대구경 거울 제조 방법이 소개되었다(Fig. 3(a))[26]. 헤테로세칼 스트레스 랩핑 기술은 불균일한 압력 분포를 보상하여 엣지 효과(Edge Effect)라고 불리우는 연마 공정의 문제점을 감소시킬 수 있다(Fig. 3(b)). 거울의 중심에서 외곽을 가공할수록 발생하는 연마 압력을 보상하기 위하여, 일반적인 가공은 측정된 형상 오차에 따라 체류 시간을 계산하는 직렬 모드(Serial Mode)를 사용하고 있다. 하지만, 직렬 모드는 CCOS 및 MRF와 같은 공정을 직렬로 계산하여 상대적으로 느리다는 단점이 있어, 서로 다른 공정들의 영향을 동시에 보상하는 병렬 계산 모델이 제안되었다(Fig. 3(c)). 이러한 방법을 통하여 최종적으로 표면 에러 15.2 nm Root Mean Square (RMS), 조도 0.8 nm RMS를 달성하였으며, 5% 이하의 클래딩(Cladding) 층두께 불균일도를 갖는 거울을 제작하였다.
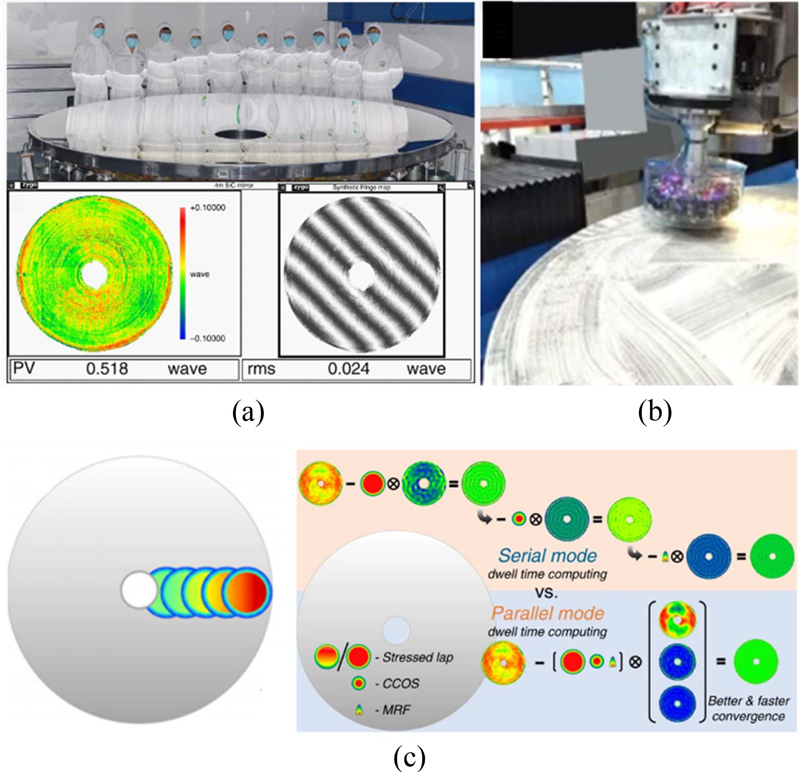
Photographs and schematics of SiC aspheric mirror manufacturing. (a) Fabrication and measurement results of the aspheric mirror. Images and illustrations of (b) heterocercal stressed lap polishing and (c) mode comparison [26] (Adapted from Ref. 26 on the basis of OA)
적층형 제조방법을 통하여 경량 거울을 제작하기 위한 다양한 방법들도 소개되었다[24,27]. 대부분의 경량 거울은 뒷면에 벌집 형태와 유사한 유닛 셀 구조를 가지고 있으며, 이 서포트 구조는 직육면체, 삼각형, 프리즘, 또는 육각 프리즘 형태 등 다양하게 설계될 수 있다. 적층형 제조방법은 다양한 형상의 서포트 구조를 구현 가능하며 이를 통하여 구조적 안정도를 향상 시킬 수 있다[28,29]. 알루미늄 합금 거울을 제작하기 위해서는 금속 선택적 레이저 용융(Selective Laser Melting, SLM) 장비를 사용하며, 레이저를 통하여 분말을 용융시켜 형상을 구성하고 분말을 제거한 후 열처리를 통하여 형상을 고정한다[30]. 적층형 제조 시 발생되는 골격(Scaffold)을 제거한 후, 형상을 보정하기 위하여 다이아몬드 정밀 선삭 가공이 추가적으로 필요하다. 그 이후 광학적 코팅을 통하여 반사도를 향상 시킨다.
2.3 반사형 광학 부품 측정
반사형 광학 부품 제조 과정에서 표면가공 정확도 및 중력등의 외부 환경에 따른 변형을 검사하기 위하여 간섭계를 통하여 표면 오차(Surface Error)와 파면 오차(Wavefront Error, WFE)를 측정한다(Fig. 4(a)) [31,32]. 표면 오차는 완벽한 표면형상 대비 편차를 관측하고, 파면 오차는 기존 파면 형상 대비 반사된 파면의 편차를 관측한다. 구면 거울의 광학 품질을 관측하기 위해서는 간섭계와 구면 거울의 초점이 같은 축에서 잘 맞도록 정렬해야 하며, 비구면 거울의 광학 품질은 일반적으로 비축 정렬을 통하여 측정한다. 중력에 의한 영향을 분석하기 위해서는 거울과 광축을 중력방향으로 맞추어 거울의 표면 변화를 관측한다[33]. 형상 오차는 또한 플로필러미터(Profilometer)를 통해서도 측정이 가능하다[34]. 접촉식 플로필러미터는 미세 캔틸레버(Microcantilever)를 샘플에 접촉 후 레이저 빔의 편차를 이용하여 형상의 프로파일을 관측한다. 비접촉식 광학 플로필러미터는 공초점 또는 홀로그래픽 방식 등을 통하여 표면의 형상 및 조도를 관측한다. 하지만 일반적인 플로필러미터는 관측 범위가 좁아 대구경광학계의 전체 표면오차를 관측하기에 어려움이 있다. 이러한 한계를 극복하고자 플로필러미터를 회전시켜가며 거울의 넓은 영역을 측정하는 스윙 암 플로필러메트리(Swing Arm Profilometry, SAP) 방법도 소개되었다(Fig. 4(b))[26,35].
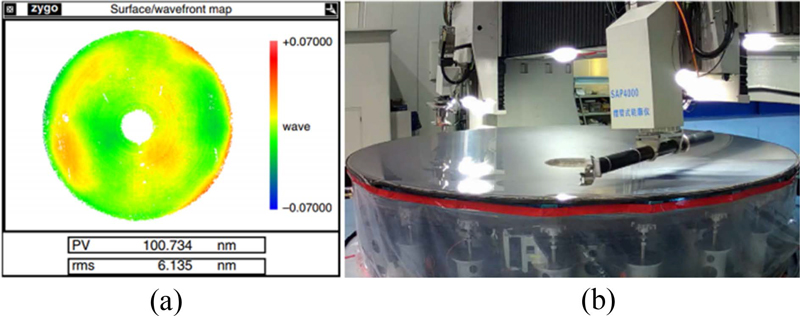
Measurement of reflective optics. (a) An image of surface error measurement through the interferometer. (b) A photograph of swing arm profilometry measurement approach [26] (Adapted from Ref. 26 on the basis of OA)
거울을 지지하는 양각대(Isostatic Bipod) 조립 및 주경과 부경의 정렬에 의한 파면 오차도 측정이 필요하다. 조립 정렬불량은 토크, 굽힘 모멘트 및 중력과 결합된 영향으로 인하여 파면 왜곡을 발생시키고, 이는 결과적으로 변조전달함수(Modulation Trasfer Function, MTF)와 같은 광학적 해상도에 영향을 준다. 파면 오차를 관측하는데 제르니케 다항식(Zernike Polynomials) 계수가 사용되며 포커스, 코마, 비점 수차와 같은 항목의 RMS 값을 분석한다[4,28]. 조립 정렬 시 제르니케 계수의 정보를 관측하며 파면 오차가 최소화되도록 틸트 및 변위를 조절한다.
3. 항공우주 광학계 응용
3.1 위성용 광학계 설계
인공위성은 무게 및 사이즈에 따라서 대형, 중형, 소형, 마이크로, 나노(or CubeSat), 피코, 펨토위성으로 분류되며, 위성의 크기와 관측 목표(Target)에 따라 광학계의 설계가 달라진다[36]. 나노위성 이상의 크기에서는 대부분 반사형 광학계를 채택하며, 카세그레인식 구조를 통하여 빛을 1차적으로 집속시킨다[37]. 관측하고자 하는 대상물의 위치, 크기뿐 아니라 관측 파장에 따라서 광학계의 설계가 달라 질 수 있다. 지구 관측용 위성은 관측 파장에 따라 기후변화, 토양수분, 해수온도 등 다양한 정보를 획득 가능하여 다양한 파장을 획득할 수 있는 광학 설계가 필요하다. 이러한 요구조건을 충족시키 위하여, 가시광(Visible) 영역의 파장과 장파장 적외선(Long Wave Infrared, LWIR)을 분리시키는 광학계 설계가 소개되었다(Fig. 5(a)) [38]. 이 광학계는 동축 반사 시스템을 통과한 시야각이 서로 다른 빔을 분리하여 흡수 파장이 서로 다른 센서에 결상되도록 설계하였다. 광학 시스템의 사이즈를 최소화하기 위하여 폴딩(Folding) 거울 구조를 통하여 빔을 집속 시켰으며, 이러한 시스템을 통하여 낮과 밤 동안 관측이 가능한 광학계를 설계하였다. 이 광학계는 가시광 파장에서 50 lp/mm (Line Pair per Millimeter)의 MTF 값 0.45를 달성 하였으며, 적외선 파장에서는 0.35 값을 보였다. 앞서 소개한 방식과 같이 광학계의 전체 공간을 최소화하기 위하여 위성용 광학계에서는 폴딩 거울 구조를 주로 사용한다. 폴딩 거울 구조를 설계하기 위해서는 거울 기울기 방향과 이미지 평면의 위치를 설정하여야 한다. 거울 기울기는 광선이 가려짐을 방지하는데 필요한 정도로 최소화해야 하며 초점거리, 시야각, 플랫 필드 등의 요구조건에 따라서 거울의 곡률 및 배치 각도 등이 변경될 수 있다[39]. Fig. 5(b)와 같이 다양한 설계 예시를 그룹별로 분류한 후 공간 제약 및 광학적 성능을 비교하여 최종 선택할 수 있다[40]. 여기서 Tier2는 공간 제약의 한계가 있으며, Tier3은 광학 성능이 우수하지 못한 설계이다. Tier4는 공간과 광학 성능 이슈가 동시에 있으며, Tier1이 가장 최적화된 설계이다.
3.2 반사형 광학계 환경 시뮬레이션
우주환경에서 임무를 수행하는 광학계는 주로 외부에 노출이 되어 있어 온도에 의한 변형, 중력 및 진동 등에 의한 분석이 필요하다[33,41]. 특히, 광학계의 형상 변화 및 내부 정렬이 틀어질 경우 광학적 성능이 저해되는 문제가 있어, 광학 허용오차 범위 내에서 형상 및 정렬을 유지시키기 위한 시뮬레이션 검증이 필요하다. 온도의 변화는 광학 표면을 변형시키거나, 열팽창의 따른 고정 부품의 변형을 야기시켜 온도변화가 클 경우 초점 변화가 나타나며 영상의 왜곡이 발생한다. 시스템이 갖는 고유 진동 및 가속에 의한 압력도 광학계에 변형을 주는 인자로 설계시 고려가 필요하다. HERA 위성이 포함하고 있는 탑재체의 변형을 분석하기 위한 연구가 소개되었다(Fig. 6(a)) [42]. HERA 위성의 광학계는 티타늄 소재의 등압 양각대에 의해 제로듀어 거울이 지지되며, 온도 변화에 따른 거울 및 고정 부품의 형상 변화를 관측하였다. 거울과 양각대 부품의 고정은 에폭시가 이용되었다. 시뮬레이션을 위하여 양각대가 볼트에 의해 바닥에 고정되어 있으며, 열이 바닥에서부터 전달되는 경계조건을 설정하였다. -60°C 및 +80°C 조건에서의 시뮬레이션을 진행한 결과 주경에서 곡률 및 XZ축 변형 등이 발생하였지만 수백 nm 수준으로 허용 오차 기준에 충족되었다(Fig. 6(b)). 열에 의하여 양각대에 전달되는 최대 응력도 안전 계수(Safety Factor)의 허용응력 기준에 충족된 결과를 보였다(Fig. 6(c)). 최근 거울과 하우징(Housing)을 동일한 소재로 제작하여 재료 차이에 의한 열팽창 차이를 제거하기 위한 구조가 많이 사용되고 있으며, 이러한 구조의 환경 시뮬레이션도 진행되었다[43].
3.3 호밍 유도 시스템
대상물을 추적하는 호밍 유도에는 신호를 직접 발생시켜 탐지하는 능동(Active) 방식, 외부에서 신호 발생시켜 수집하는 반능동(Semi-active) 방식, 표적에서 발생하는 신호를 획득하는 수동(Passive) 방식이 있다[44]. 탐지형 호밍 유도시스템은 대상물의 열에서 방출되는 적외선을 추적하는 능동형 방식을 주로 이용한다[45]. 호밍 유도 시스템은 위성용 광학계와 달리 주로 항공기 외부에 부착이 되어 외부 날씨나 유체역학적 응력으로부터 광학계를 보호하기 위하여 적외선 투과 돔이 주로 설치된다[46]. 광학계 구성은 위성용 광학계와 유사하게 주경과 부경을 포함하는 반사형 타입을 이용하며, 반사된 빔은 레티클을 통과하여 센서로 전달된다. 레티클은 광 변조의 목적으로 장착이 되며, 레티클의 투명하고 불투명한 배열과 간격에 따라서 다른 주파수를 획득된다. 대상물의 위치에 따라 뉴테이션 원(Nutation Circle)의 위치가 달라지고, 지속적으로 광학계 구경의 위치를 조정하며 대상물을 추적한다[47,48]. 레티클이 회전하는 경우 진폭변조(Amplitude Modulation, AM)을 통하여 신호를 처리하고, 레티클이 고정되어 있는 경우 주파수 변조(Frequency Modulation, FM)을 통하여 신호를 획득한다(Fig. 7(a)) [49]. 광기구적으로 적외선 유도 광학계를 스캐닝하여 대상물을 추적하는 구조는 대상물을 지속적으로 추적하는데 도움을 준다(Figs. 7(b), 7(c)) [12]. 스캐닝 기구물 구조로는 자이로 로터(Gyro Rotor), 구동모터(Control Motor), 각도 보정 광섬유 센서(Fibreoptical Sensor), 하우징 등이 있다. 고정되어 있는 외부 하우징과 움직임이 있는 내부 하우징의 좌표축의 차이를 지속적으로 수정하여 회전 각도를 계산한다.
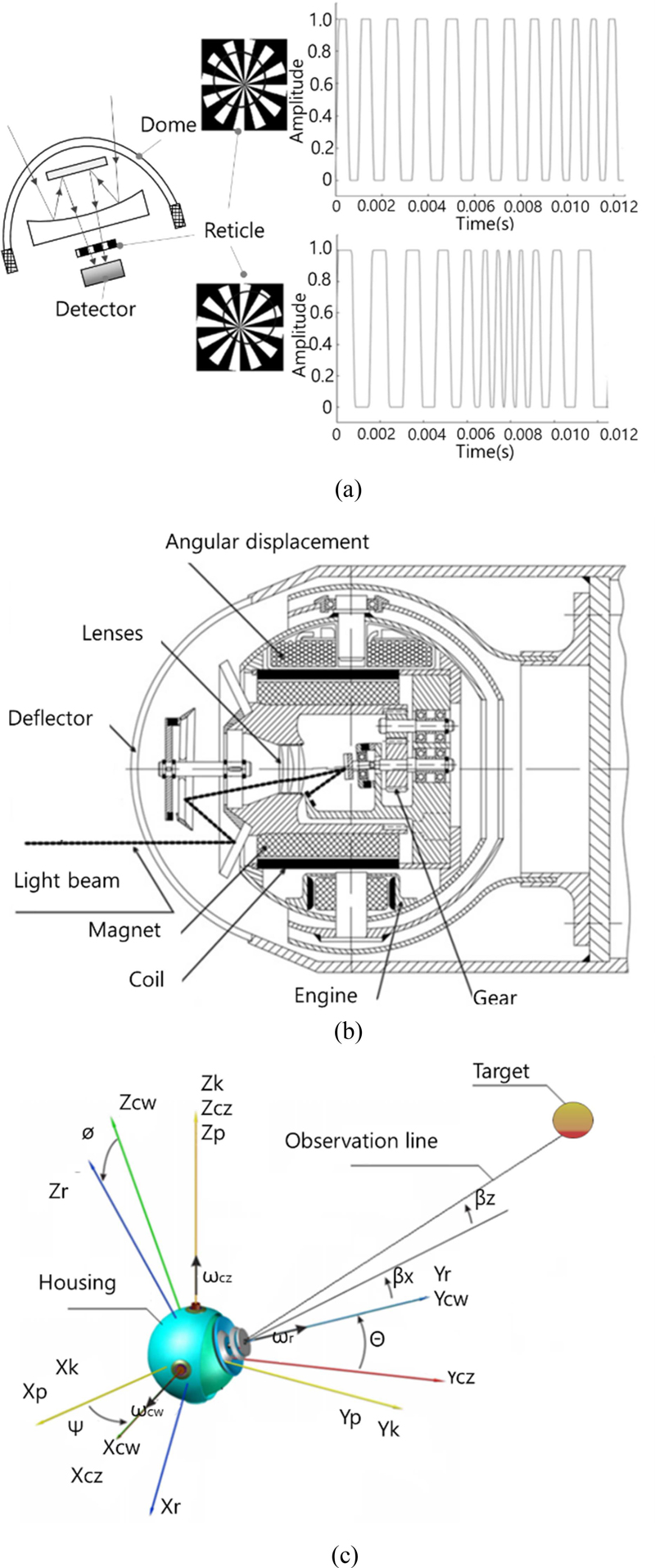
Principles and configurations of homing optical systems. (a) Principles of tracking error signal extraction using a reticle [49] (Adapted from Ref. 49 on the basis of OA) (b) A schematic of a scanning homing system structure. (c) Tracking diagram with an established coordinate [12] (Adapted from Ref. 12 on the basis of OA)
4. 결론
본 논문에서는 항공우주 기술에 활용되는 반사형 광학 시스템에 대하여 소개를 하였다. 반사형 광학계는 지구 및 우주 관측용 위성뿐 아니라 호밍유도를 위한 추적 시스템에 사용되고 있다. 반사형 광학 부품은 주로 세라믹, 유리 및 금속 재료를 통하여 제작이 되며 비용, 열안정성 및 기계적 특성에 따라 소재를 선택해야 한다. 반사형 광학부품을 제작하는 방법은 크게 기계적 가공과 적측형 제조로 구분이 되며, 적층형 제조 방법은 경량화 구조를 제작하는데 장점이 있으나 소재의 한계로 인하여 열안정성이 높은 광학부품 제작이 어렵다는 단점이 있다. 위성용 광학계의 경우 주경과 부경이 포함된 카세그리안식 형태를 주로 채택하며, 환경 시뮬레이션을 통하여 광학적 특성 변화를 예측한다. 호밍 유도 시스템에도 반사형 광학계가 주요 부품으로 장착이 되며, 레티클을 통과한 신호를 분석하여 대상물의 위치를 실시간으로 파악한다. 반사형 광학계의 경우 크기가 커질수록 높은 성능을 구현할 수 있어 시스템 크기도 지속적으로 커질 것으로 예상된다. 특히, 우주 망원경의 경우 원거리 이미징을 위하여 대형 광학계가 요구되어지고 있으나 발사체 공간의 한계가 있어 전개형 반사 광학계를 주로 채택한다. 광학계 전개 시 발생할 수 있는 여러 가지 환경 및 정렬 오차에 의한 변형들은 광학 성능을 결정하는 주요 요소이기 때문에 외부 환경조건에 따른 광학성능을 사전에 예측하는 연구의 중요성이 증가할 것으로 예상된다. 또한, 항공우주 응용분야의 효율적인 임무 수행을 위하여 여러 가지 파장을 동시에 관측하는 초분광 이미징용 반사형 광학부품에 대한 공정 및 분광 측정 기술도 지속적으로 발전할 것으로 예상된다.
Acknowledgments
This work was supported by the Agency for Defense Development Grant Funded by the Korean Government (UI230005SD).
References
-
Lightsey, P. A., Atkinson, C., Clampin, M., Feinberg, L. D., (2012), James webb space telescope: Large deployable cryogenic telescope in space, Optical Engineering, 51(1), 011003-011003.
[https://doi.org/10.1117/1.OE.51.1.011003]
-
Rigby, J., Perrin, M., McElwain, M., Kimble, R., Friedman, S., Lallo, M., Doyon, R., Feinberg, L., Ferruit, P., Glasse, A., (2023), The science performance of JWST as characterized in commissioning, Publications of the Astronomical Society of the Pacific, 135(1046), 048001.
[https://doi.org/10.1088/1538-3873/acbcf4]
-
Zhang, C., Li, Z., (2022), A review of lightweight design for space mirror core structure: Tradition and future, Machines, 10(11), 1066.
[https://doi.org/10.3390/machines10111066]
-
Qu, Y., Jiang, Y., Feng, L., Li, X., Liu, B., Wang, W., (2018), Lightweight design of multi-objective topology for a large-aperture space mirror, Applied Sciences, 8(11), 2259.
[https://doi.org/10.3390/app8112259]
-
Wu, J., (2020), Design of high-lightweight space mirror component based on automatic optimization, Journal of Physics: Conference Series, 012023.
[https://doi.org/10.1088/1742-6596/1605/1/012023]
-
Wu, H.-Y., Chang, W.-C., Huang, P.-H., Huang, Y.-K., (2019), New candidate substrate materials for space mirror optics, Material Technologies and Applications to Optics, Structures, Components, and Sub-Systems IV, 157-161.
[https://doi.org/10.1117/12.2530689]
-
Zhang, Y., Liu, D., Zheng, Z., Liu, Z., Hu, D., Qi, B., Liu, C., Bi, L., Zhang, K., Wen, C., (2018), Effects of auxiliary atmospheric state parameters on the aerosol optical properties retrieval errors of high-spectral-resolution lidar, Applied Optics, 57(10), 2627-2637.
[https://doi.org/10.1364/AO.57.002627]
-
Hou, Y., Wang, C., Duan, P., Yu, H., Zhao, X., Chen, S., Zhang, L., (2023), A general method to obtain the frequency response function from the disturbance source to the sensitive payload, Applied Sciences, 13(8), 4844.
[https://doi.org/10.3390/app13084844]
-
Kazasidis, O., Verpoort, S., Wittrock, U., (2019), Image-based wavefront correction for space telescopes, Proceedings of the International Conference on Space optics-ICSO 2018, 11180, 2854-2866.
[https://doi.org/10.1117/12.2536206]
-
Abbas, O., Arvan, M. R., Mahmoodi, A., (2020), Real time extraction of tracking error signal in IR seeker with a plus stationary reticle, Infrared Physics & Technology, 104, 103145.
[https://doi.org/10.1016/j.infrared.2019.103145]
-
Ocokoljić, G. J., Rašuo, B. P., Bengin, A. Č., (2017), Aerodynamic shape optimization of guided missile based on wind tunnel testing and computational fluid dynamics simulation, Thermal Science, 21(3), 1543-1554.
[https://doi.org/10.2298/TSCI150515184O]
-
Gapiński, D., Koruba, Z., (2021), Control of optoelectronic scanning and tracking seeker by means the LQR modified method with the input signal estimated using of the extended kalman filter, Energies, 14(11), 3109.
[https://doi.org/10.3390/en14113109]
-
Zhu, M., Chen, H., Xiong, G., (2017), A model predictive speed tracking control approach for autonomous ground vehicles, Mechanical Systems and Signal Processing, 87, 138-152.
[https://doi.org/10.1016/j.ymssp.2016.03.003]
-
Guo, H., Cao, D., Chen, H., Sun, Z., Hu, Y., (2019), Model predictive path following control for autonomous cars considering a measurable disturbance: Implementation, testing, and verification, Mechanical Systems and Signal Processing, 118, 41-60.
[https://doi.org/10.1016/j.ymssp.2018.08.028]
-
Steinkopf, R., Gebhardt, A., Scheiding, S., Rohde, M., Stenzel, O., Gliech, S., Giggel, V., Löscher, H., Ullrich, G., Rucks, P., (2008), Metal mirrors with excellent figure and roughness, Optical Fabrication, Testing, and Metrology III, 162-173.
[https://doi.org/10.1117/12.797702]
-
Ji, S., Yu, H., Zhao, J., Liu, X., Zhao, M., (2016), Ultra-precision machining of a large amplitude sinusoidal ring surface based on a slow tool servo/Ultranatancna obdelava sinusoidne povrsine obrocev z veliko amplitudo po tehnologiji Slow Tool Servo, Strojniski Vestnik-Journal of Mechanical Engineering, 62(4), 213-221.
[https://doi.org/10.5545/sv-jme.2015.2528]
-
Vukobratovich, D., Schaefer, J. P., (2011), Large stable aluminum optics for aerospace applications, Optomechanics 2011: Innovations and Solutions, 260-272.
[https://doi.org/10.1117/12.892039]
-
Du, C., Dai, Y., Guan, C., Hu, H., (2021), High efficiency removal of single point diamond turning marks on aluminum surface by combination of ion beam sputtering and smoothing polishing, Optics Express, 29(3), 3738-3753.
[https://doi.org/10.1364/OE.417537]
-
Li, L., Yu, N., Chan, C., Lee, W., (2018), Al6061 surface roughness and optical reflectance when machined by single point diamond turning at a low feed rate, Plos One, 13(4), e0195083.
[https://doi.org/10.1371/journal.pone.0195083]
-
Sampath, D., Akerstrom, A., Barry, M., Guregian, J., Schwalm, M., Ugolini, V., (2010), The WISE telescope and scanner: design choices and hardware results, An Optical Believe It or Not: Key Lessons Learned II, 79-89.
[https://doi.org/10.1117/12.864347]
-
Gardner, J. P., Mather, J. C., Clampin, M., Doyon, R., Greenhouse, M. A., Hammel, H. B., Hutchings, J. B., Jakobsen, P., Lilly, S. J., Long, K. S., (2006), The james webb space telescope, Space Science Reviews, 123, 485-606.
[https://doi.org/10.1007/s11214-006-8315-7]
-
Lee, J.-H., Lee, C.-W., Kim, Y.-M., Kim, J.-W., (2009), Optomechanical design of a compact imaging spectrometer for a microsatellite STSAT3, Journal of the Optical Society of Korea, 13(2), 193-200.
[https://doi.org/10.3807/JOSK.2009.13.2.193]
- Pawar, P., Ballav, R., Kumar, A., (2017), Machining processes of silicon carbide: A review, Review on Advanced Materials Science, 51, 62-76.
-
Zhang, K., Qu, H., Guan, H., Zhang, J., Zhang, X., Xie, X., Yan, L., Wang, C., (2021), Design and fabrication technology of metal mirrors based on additive manufacturing: A review, Applied Sciences, 11(22), 10630.
[https://doi.org/10.3390/app112210630]
-
Jalluri, T. D., Rao, B. V., Rudraswamy, B., Venkateswaran, R., Sriram, K., (2023), Optical polishing and characterization of chemical vapour deposited silicon carbide mirrors for space applications, Journal of Optics, 52(3), 969-983.
[https://doi.org/10.1007/s12596-022-00925-w]
-
Zhang, X., Hu, H., Wang, X., Luo, X., Zhang, G., Zhao, W., Wang, X., Liu, Z., Xiong, L., Qi, E., Cui, C., Wang, Y., Li, Y., Wang, X., Li, L., Bai, Y., Cheng, Q., Zhang, Z., Li, R., Tang, W., Zeng, X., Deng, W., Zhang, F., (2022), Challenges and strategies in high-accuracy manufacturing of the world’s largest SiC aspheric mirror, Light: Science & Applications, 11(1), 310.
[https://doi.org/10.1038/s41377-022-00994-3]
-
Fan, Y., Dong, D., Li, C., Sun, Y., Zhang, Z., Wu, F., Yang, L., Li, Q., Guan, Y., (2021), Research and experimental verification on topology-optimization design method of space mirror based on additive-manufacturing technology, Machines, 9(12), 354.
[https://doi.org/10.3390/machines9120354]
-
Snell, R., Atkins, C., Schnetler, H., Todd, I., Hernández-Nava, E., Lyle, A. R., Maddison, G., Morris, K., Miller, C., Roulet, M., (2020), An additive manufactured CubeSat mirror incorporating a novel circular lattice, Advances in Optical and Mechanical Technologies for Telescopes and Instrumentation IV, 58-73.
[https://doi.org/10.1117/12.2562738]
-
Schneider, J., Norman, A., Gumpinger, J., Brückner, F., Bavdaz, M., Leyens, C., Ghidini, T., (2023), Additive manufacturing of a metallic optical bench—process development, material qualification and demonstration, CEAS Space Journal, 15(1), 55-68.
[https://doi.org/10.1007/s12567-021-00409-w]
-
Hilpert, E., Hartung, J., von Lukowicz, H., Herffurth, T., Heidler, N., (2019), Design, additive manufacturing, processing, and characterization of metal mirror made of aluminum silicon alloy for space applications, Optical Engineering, 58(9), 092613-092613.
[https://doi.org/10.1117/1.OE.58.9.092613]
-
Malacara, D., (2007), Optical shop testing, John Wiley & Sons.
[https://doi.org/10.1002/9780470135976]
-
Zhang, H., Liu, H., Lizana, A., Xu, W., Caompos, J., Lu, Z., (2017), Methods for the performance enhancement and the error characterization of large diameter ground-based diffractive telescopes, Optics Express, 25(22), 26662-26677.
[https://doi.org/10.1364/OE.25.026662]
-
Tan, S., Zhang, X., Wang, L., Wu, H., Fu, Q., Yan, L., Hu, M., (2022), Equivalent modeling and verification of a high-steepness and lightweight elliptical aluminum mirror, Applied Sciences, 12(18), 9091.
[https://doi.org/10.3390/app12189091]
-
Tsutsumi, H., Yoshizumi, K., Takeuchi, H., (2005), Ultrahighly accurate 3D profilometer, Optical Design and Testing II, 387-394.
[https://doi.org/10.1117/12.573774]
- Anderson, D. S., Burge, J. H., (1995), Swing-arm profilometry of aspherics, Optical Manufacturing and Testing, 169-179.
- Radhakrishnan, R., Edmonson, W. W., Afghah, F., Chenou, J., Rodriguez-Osorio, R. M., Zeng, Q.-A., (2014), Optimal multiple access protocol for inter-satellite communication in small satellite systems, 4S Small Satellite Systems and Services Symposium, 1-15.
-
Kerstel, E., Gardelein, A., Barthelemy, M., Fink, M., Joshi, S. K., Ursin, R., (2018), Nanobob: A CubeSat mission concept for quantum communication experiments in an uplink configuration, EPJ Quantum Technology, 5(1), 6.
[https://doi.org/10.1140/epjqt/s40507-018-0070-7]
-
Li, R., Zou, G., Feng, L., Fan, X., (2021), Design of a dual-band compact integrated remote sensing system for visible light and long-wave infrared, Applied Sciences, 11(20), 9370.
[https://doi.org/10.3390/app11209370]
-
Zhang, B., Jin, G., Zhu, J., (2021), Towards automatic freeform optics design: Coarse and fine search of the three-mirror solution space, Light: Science & Applications, 10(1), 65.
[https://doi.org/10.1038/s41377-021-00510-z]
-
Bauer, A., Schiesser, E. M., Rolland, J. P., (2018), Starting geometry creation and design method for freeform optics, Nature Communications, 9(1), 1756.
[https://doi.org/10.1038/s41467-018-04186-9]
-
Jeong, S., Nam, J. W., Ahn, K. B., Park, I. H., Kim, S. W., Lee, J., Lim, H., Brandt, S., Budtz-Jørgensen, C., Castro-Tirado, A. J., Chen, P., Cho, M. H., Choi, J. N., Grossan, B., Huang, M. A., Jung, A., Kim, J. E., Kim, M. B., Kim, Y. W., Linder, E. V., Min, K. W., Na, G. W., Panasyuk, M. I., Ripa, J., Reglero, V., Smoot, G. F., Suh, J. E., Svertilov, S., Vedenkin, N., Yashin, I., (2013), Slewing mirror telescope optics for the early observation of UV/optical photons from gamma-ray bursts, Optics Express, 21(2), 2263-2278.
[https://doi.org/10.1364/OE.21.002263]
-
Dias, N. G., Gordo, P., Onderwater, H., Melicio, R., Amorim, A., (2022), Analysis on the isostatic bipod mounts for the HERA mission LIDAR, Applied Sciences, 12(7), 3497.
[https://doi.org/10.3390/app12073497]
-
Peschel, T., Damm, C., Beier, M., Gebhardt, A., Risse, S., Walter, I., Sebastian, I., Krutz, D., (2017), Design of an imaging spectrometer for earth observation using freeform mirrors, Proceedings of the International Conference on Space Optics—ICSO 2016, 928-936.
[https://doi.org/10.1117/12.2296156]
- Palumbo, N. F., (2010), Guest editor’s introduction: Homing missile guidance and control, John Hopkins APL Technical Digest, 29(1), 2-8.
-
Pandey, D., Wairya, S., Sharma, M., Gupta, A. K., Kakkar, R., Pandey, B. K., (2022), An approach for object tracking, categorization, and autopilot guidance for passive homing missiles, Aerospace Systems, 5(4), 553-566.
[https://doi.org/10.1007/s42401-022-00150-0]
-
Kakkar, R., Singh, S., Singh, J., Goyal, S., Khosla, D., Sharma, M., (2021), Investigations on mathematical modeling of imaging infrared (IIR) missile, Recent Innovations in Computing: Proceedings of International Conference Recent Innovations in Computing 2020, 381-390.
[https://doi.org/10.1007/978-981-15-8297-4_31]
-
Bae, T.-W., Kim, B.-I., Kim, Y.-C., Ahn, S.-H., (2012), Jamming effect analysis of infrared reticle seeker for directed infrared countermeasures, Infrared Physics & Technology, 55(5), 431-441.
[https://doi.org/10.1016/j.infrared.2012.05.001]
-
Figen, Z. G., (2019), Mid-infrared laser source for testing jamming code effectiveness in the field, Optical Engineering, 58(8), 086101.
[https://doi.org/10.1117/1.OE.58.8.086101]
- Abbas, O., Arvan, M. R., Mahmoudi, A., (2018), Improving the tracking error signal extraction in IR seeker with stationary wagon wheel reticle over all field of view, Journal of Electrical and Computer Engineering Innovations (JECEI), 6(2), 151-158.
Received his Ph.D. degree in the Department of Bio and Brain Engineering from Korea Advanced Institute of Science and Technology (KAIST), in 2021. Now, he is a senior researcher at Korea Photonics Technology Institute (KOPTI). His research interests include optical module design and evaluation for arrayed camera systems.
E-mail: kisookim@kopti.re.kr
Received his Ph.D. degree in the School of Mechanical Engineering from Chung-Ang University, in 2018. Now, he is a senior researcher at Agency for Defense Development (ADD). His research interests include EO/IR systems.
E-mail: ghunb16@add.re.kr
Received his Ph.D. degree in the Department of Mechatronics Engineering from Gwangju Institute of Science and Technology (GIST), in 2009. Now, he is a director of Intelligent Optical Module Research Center at Korea Photonics Technology Institute (KOPTI). His research interests include optical system design for laser and imaging optic system.
E-mail: jb.park@kopti.re.kr